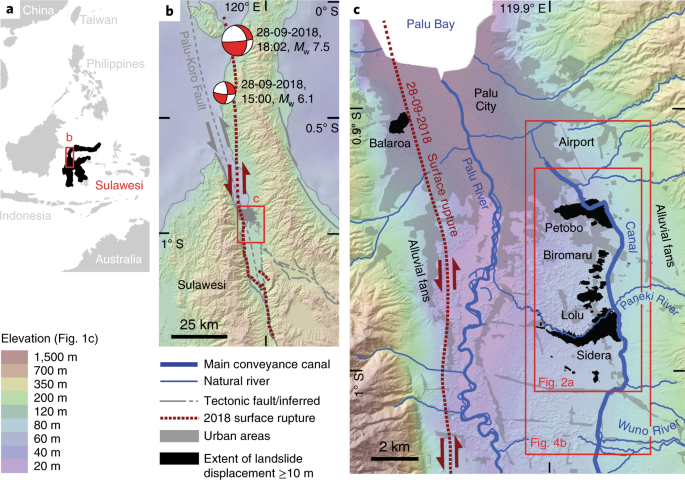
Bilham, R. Lessons from the Haiti earthquake. Nature 463, 878–879 (2010).
Holzer, L. T. & Savage, J. C. Global earthquake fatalities and population. Earthq. Spectra 29, 155–175 (2013).
Marano, K. D., Wald, D. J. & Allen, T. I. Global earthquake casualties due to secondary effects: a quantitative analysis for improving rapid loss analyses. Nat. Hazards 52, 319–328 (2010).
Alcántara-Ayala, I., Esteban-Chávez, O. & Parrot, J. F. Landsliding related to land-cover change: a diachronic analysis of hillslope instability distribution in the Sierra Norte, Puebla, Mexico. Catena 65, 152–165 (2006).
Pisano, L. et al. Variations in the susceptibility to landslides, as a consequence of land cover changes: a look to the past, and another towards the future. Sci. Total Environ. 601–602, 1147–1159 (2017).
Sangelantoni, L., Gioia, E. & Marincioni, F. Impact of climate change on landslides frequency: the Esino river basin case study (Central Italy). Nat. Hazards 93, 849–884 (2018).
Barnard, P. L., Owen, L. A., Sharma, M. C. & Finkel, R. C. Natural and human-induced landsliding in the Garhwal Himalaya of northern India. Geomorphology 40, 21–35 (2001).
Hearn, G. J. & Shakya, N. M. Engineering challenges for sustainable road access in the Himalayas. Q. J. Eng. Geol. Hydrogeol. 50, 69–80 (2017).
Zhang, D., Wang, D., Luo, C., Chen, J. & Zhou, Y. A rapid loess flowslide triggered by irrigation in China. Landslides 6, 55–60 (2009).
Tanyas, H. et al. Presentation and analysis of a worldwide database of earthquake-induced landslideinventories. J. Geophys. Res. Earth Surf. 122, 1991–2015 (2017).
Owen, L. A. et al. Landslides triggered by the October 8, 2005, Kashmir earthquake. Geomorphology 94, 1–9 (2008).
Keefer, D. K. Investigating landslide caused by earthquakes—a historical review. Surv. Geophys. 23, 473–510 (2002).
Bellier, O. et al. High slip rate for a low seismicity along the Palu-Koro active fault in central Sulawesi (Indonesia). Terra Nova 13, 463–470 (2001).
Socquet, A. et al. Microblock rotations and fault coupling in SE Asia triple junction (Sulawesi, Indonesia) from GPS and earthquake slip vector data. J. Geophys. Res. 111, B08409 (2006).
Thein, P. S. et al. Site response characteristics of H/V spectrum by microtremor single station observations at Palu city, Indonesia. J. SE Asian Appl. Geol. 5, 1–9 (2013).
Cipta, A. et al. in Geohazards in Indonesia: Earth Science for Disaster Risk Reduction (eds Cummins, P. R. & Meilano, I.) 133–152 (Geological Society, 2017).
Watkinson, I. M. & Hall, R. in Geohazards in Indonesia: Earth Science for Disaster Risk Reduction (eds Cummins, P. R. & Meilano, I.) 71–120 (Geological Society, 2017).
Metzner, J. Palu(Sulawesi) Palu (Sulawesi) problems of land utilisation in a climatic dry valley on the equator. Erdkunde 35, 42–54 (1981).
Pelinovsky, E., Yuliadi, D., Prasetya, G. & Hidayat, R. The 1996 Sulawesi tsunami. Nat. Hazards 16, 29–38 (1997).
Sutapa, I. W. & Galib, I. M. Application of non-parametric test to detect trend rainfall in Palu watershed, Central Sulawesi, Indonesia. Int. J. Hydrol. Sci. Technol. 6, 238–253 (2016).
Socquet, A., Hollingsworth, J., Pathier, E. & Bouchon, M. Evidence of supershear during the 2018 magnitude 7.5 Palu earthquake from space geodesy. Nat. Geosci. 12, 192–199 (2019).
Bao, H. et al. Early and persistent supershear rupture of the 2018 magnitude 7.5 Palu earthquake. Nat. Geosci. 12, 200–205 (2019).
Situation Update No.15—Final. M7.4 Earthquake & Tsunami Sulawesi, Indonesia (ASEAN Coordinating Centre for Humanitarian Assistance on Disaster Management, accessed 25 November 2018); https://reliefweb.int/report/indonesia/aha-centre-situation-update-no-15-final-m-74-earthquake-and-tsunami-sulawesi
Weber, R., Kreisel, W. & Faust, H. Colonial Interventions on the cultural landscape of Central Sulawesi by “ethical policy”: the impact of the Dutch rule in Palu and Kulawi valley, 1905–1942. Asian J. Soc. Sci. 31, 398–434 (2003).
Keil, A., Zeller, M., Wida, A., Sanim, B. & Birner, R. What determines farmers’ resilience towards ENSO-related drought? An empirical assessment in Central Sulawesi, Indonesia. Clim. Change 86, 291–307 (2008).
Hamilton, W. in Professional Paper 1078 (US Geological Survey, 1979).
Dunbar, P. K., Lockridge, P. A. & Whiteside, L. S. Catalog of Significant Earthquakes 2150 B.C.–1991 A.D (National Geophysical Data Center, 1992).
Hamzah, L., Puspito, N. T. & Imamura, F. Tsunami catalog and zones in Indonesia. J. Nat. Disaster Sci. 22, 25–43 (2000).
Prasetya, G. S., de Lange, W. P. & Healy, T. R. The Makassar Strait tsunamigenic region, Indonesia. Nat. Hazards 24, 295–307 (2001).
Varnes, D. J. in Landslides, Analysis and Control Special Report 176 (eds Schuster, R. L. & Krizek, R. J.) 11–33 (Transport Research Board, National Academy of Sciences, 1978).
Youd, T. L. & Garris, C. T. Liquefaction-induced ground-surface disruption. J. Geotechnol. Eng. 121, 805–809 (1995).
Bartlett, S. F. & Youd, T. L. Empirical Analysis of Horizontal Ground Displacement Generated by Liquefaction-induced Lateral Spreads (National Centre for Earthquake Research, 1992).
Glass, C. E. Interpreting Aerial Photographs to Identify Natural Hazards (Elsevier, 2013).
Imtiyaz A. Parvez & Rosset, P. in Earthquake Hazard, Risk and Disasters (ed. Wyss, M.) 273–304 (Elsevier, 2014).
Youd, L. T. in International Handbook of Earthquake and Engineering Seismology (eds Lee, W. H. K. et al.) 1159–1173 (Academic Press, 2003).
Sukamto, R. et al. Reconnaissance Geological Map of the Palu Quadrangle, Sulawesi (Geological Research and Development Centre, 1973).
van Leeuwen, T. M. & Muhardjo Stratigraphy and tectonic setting of the Cretaceous and Paleogene volcanic–sedimentary successions in northwest Sulawesi, Indonesia: implications for the Cenozoic evolution of Western and Northern Sulawesi. J. Asian Earth Sci. 25, 481–511 (2005).
Iverson, R. M. et al. Landslide mobility and hazards: implications of the 2014 Oso disaster. Earth Planet. Sci. Lett. 412, 197–208 (2015).
Moayedi, H. et al. Preventing landslides in times of rainfall: case study and FEM analyses. Disaster Prev. Manag. 20, 115–124 (2011).
Bolton Seed, H. & Wilson, S. D. The Turnagain Heights landslide, Anchorage. Alask. J. Soil Mech. Found. Div. 93, 325–353 (1967).
Derbyshire, E., Meng, X. M. & Dijkstra, T. A. Landslides in the Thick Loess Terrain of North-West China (Wiley, Chichester, 2000).
Ishihara, K. et al. Geotechnical aspects of the June 20, 1990 Manjil earthquake in Iran. Soils Found. 32, 61–78 (1992).
Evans, S. G. et al. Landslides triggered by the 1949 Khait earthquake, Tajikistan, and associated loss of life. Eng. Geol. 109, 195–212 (2009).
Ishihara, K., Okusa, S., Oyagi, N. & Ischuk, A. Liquefaction-induced flow slide in the collapsible loess deposit in Soviet Tajik. Soils Found. 30, 73–89 (1990).
Sato, S., Yamaji, E. & Kuroda, T. Strategies and engineering adaptions to disseminate SRI methods in large-scale irrigation systems in Eastern Indonesia. Paddy Water Environ. 9, 79–88 (2011).
Naing, M. M. in Proceedings of the Regional Workshop on the Future of Large Rice-Based Irrigation Systems in Southeast Asia 120–130 (Vietnam Institute for Water Resources Research, 2005).
Mukherji, A. et al. Revitalizing Asia’s Irrigation: To Sustainably Meet Tomorrow’s Food Needs (International Water Management Institute & Food and Agriculture Organization of the United Nations, 2009).
201809281002AMinahassa Peninsula, SUL (Global Centroid-Moment-Tensor Project, accessed 25 November 2018); https://www.globalcmt.org/
Dziewonski, A. M., Chou, T.-A. & Woodhouse, J. H. Determination of earthquake source parameters from waveform data for studies of global and regional seismicity. J. Geophys. Res. 86, 2825–2852 (1981).
Ekström, G., Nettles, M. & Dziewonski, A. M. The global CMT project 2004–2010: centroid-moment tensors for 13,017 earthquakes. Phys. Earth Planet. Inter. 200–201, 1–9 (2012).
Digital Globe (Digital Globe, 2018); https://go.nature.com/2lY2Xpx
Sheppard, S. R. J. & Cizek, P. The ethics of Google Earth: crossing thresholds from spatial data to landscape visualisation. J. Environ. Manag. 90, 2102–2117 (2009).
Sentinel Online (ESA, 2018); https://sentinel.esa.int/web/sentinel/missions/sentinel-2
Hajnsek, I. et al. TanDEM-X: TanDEM-X Digital Elevation Models Announcement of Opportunity; TD-PD-AO-0033 (German Aerospace Center, Microwaves and Radar Institute, 2016).
Potere, D. Horizontal positional accuracy of Google Earth’s high-resolution imagery archive. Sensors 8, 7973–7981 (2008).
Mohammed, N. Z., Ghazi, A. & Mustafa, H. E. Positional accuracy testing of Google Earth. Int. J. Multidiscipl. Sci. Eng. 4, 6–9 (2013).
Pulighe, G., Baiocchi, V. & Lupia, F. Horizontal accuracy assessment of very high resolution Google Earth images in the city of Rome, Italy. Int. J. Digit. Earth 9, 342–362 (2016).
Benker, S. C., Langford, R. P. & Pavilis, T. L. Positional accuracy of the Google Earth terrain model derived from stratigraphic unconformities in the Big Bend region, Texas, USA. Geocarto Int. 26, 1–13 (2011).
Youssef, A. M., Maerz, N. H. & Hassan, A. M. Remote sensing applications to geological problems in Egypt: case study, slope instability investigation, Sharm El-Sheikh/Ras-Nasrani area, southern Sinai. Landslides 6, 353–360 (2009).
Stumpf, A., Lampert, T. A., Malet, J.-P. & Kerle, N. Multi-scale line detection for landslide fissure mapping. In Proc. IEEE International Geoscience and Remote Sensing Symposium (IEEE, 2012).
Parise, M. Observation of surface features on an active landslide, and implications for understanding its history of movement. Nat. Hazards Earth Syst. Sci. 3, 569–580 (2003).
Stumpf, A., Malet, J.-P., Kerle, N., Niethammer, U. & Rothmund, S. Image-based mapping of surface fissures for the investigation of landslide dynamics. Geomorphology 186, 12–27 (2013).
Fleming, R. W. & Johnson, A. M. Structures associated with strike-slip faults that bound landslide elements. Eng. Geol. 27, 39–114 (1989).
Krauskopf, K. B., Feitler, S. & Griggs, A. B. Structural features of a landslide near Gilroy, California. J. Geol. 47, 630–648 (1939).
Stumpf, A., Malet, J.-P., Puissant, A. & Travelletti, J. in Land Surface Remote Sensing and Risks (eds Baghdadi, N. & Zribi, M.) 147–190 (Elsevier, 2016).
Avouac, J.-P., Ayoub, F., Leprince, S., Konca, O. & Helmberger, D. V. The 2005 Mw 7.6 Kashmir earthquake: sub-pixel correlation of ASTER images and seismic waveforms analysis. Earth Planet. Sci. Lett. 249, 514–528 (2006).
Tamkuan, N. & Nagai, M. Fusion of multi-temporal interferometric coherence and optical image data for the 2016 Kumamoto earthquake damage assessment. ISPRS Int. J. Geoinfo. 6, 188 (2017).
Sims, J. D. & Garvin, C. D. Recurrent liquefaction induced by the 1989 Loma Prieta earthquake and 1990 and 1991 aftershocks: implications for paleoseismicity studies. Bull. Seismol. Soc. Am. 85, 51–65 (1995).
Cubrinovski, M. et al. Liquefaction effects and associated damages observed at the Wellington CenrePort from the 2016 Kaikoura earthquake. Bull. N. Z. Soc. Earthq. Eng. 50, 152–173 (2017).
Quigley, M. C., Bastin, S. & Bradley, B. A. Recurrent liquefaction in Christchurch, New Zealand, during the Canterbury earthquake sequence. Geology 41, 419–422 (2013).
Wotherspoon, L. M., Pender, M. J. & Orense, R. P. Relationship between observed liquefaction at Kaiapoi following the 2010 Darfield earthquake and former channels of the Waimakariri River. Eng. Geol. 125, 45–55 (2012).
Cubrinovski, M. et al. Soil liquefaction effects in the Central Business District during the February 2011 Christchurch Earthquake. Seis. Res. Lett. 82, 893–904 (2011).
Hotelling, H. Analysis of a complex of statistical variables into principal components. J. Educ. Psych. 24, 417–441 (1933).
Sharma, S. K., Gajbhiye, S. & Tignath, S. Application of principal component analysis in grouping geomorphic parameters of a watershed for hydrological modelling. Appl. Water Sci. 5, 89–96 (2015).
Qi, J. et al. A modified soil adjusted vegetation index. Remote. Sens. Environ. 48, 119–126 (1994).
Source: Resources - nature.com