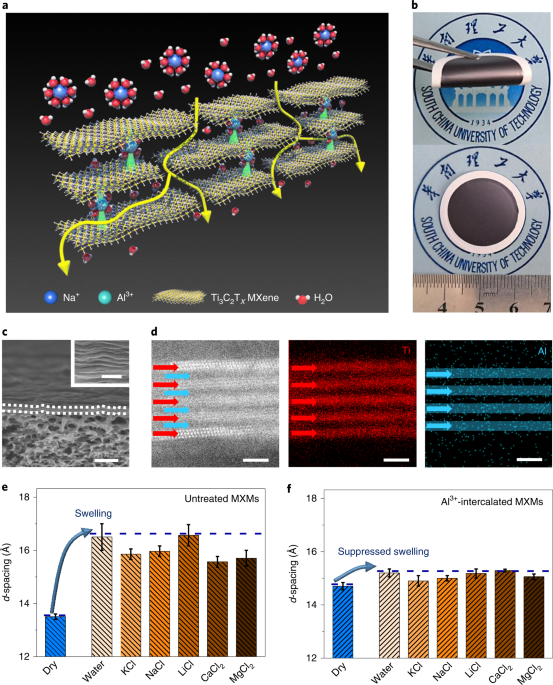
Elimelech, M. & Phillip, W. A. The future of seawater desalination: energy, technology, and the environment. Science 333, 712–717 (2011).
Van der Bruggen, B. & Vandecasteele, C. Distillation vs. membrane filtration: overview of process evolutions in seawater desalination. Desalination 143, 207–218 (2002).
Sholl, D. S. & Lively, R. P. Seven chemical separations to change the world. Nature 532, 435–437 (2016).
Van der Bruggen, B., Mänttäri, M. & Nyström, M. Drawbacks of applying nanofiltration and how to avoid them: a review. Sep. Purif. Technol. 63, 251–263 (2008).
Joshi, R. K. et al. Precise and ultrafast molecular sieving through graphene oxide membranes. Science 343, 752–754 (2014).
Mi, B. Graphene oxide membranes for ionic and molecular sieving. Science 343, 740–742 (2014).
Liu, G., Jin, W. & Xu, N. Two-dimensional-material membranes: a new family of high-performance separation membranes. Angew. Chem. Int. Ed. Engl. 55, 13384–13397 (2016).
Han, Y., Xu, Z. & Gao, C. Ultrathin graphene nanofiltration membrane for water purification. Adv. Funct. Mater. 23, 3693–3700 (2013).
Hirunpinyopas, W. et al. Desalination and nanofiltration through functionalized laminar MoS2 membranes. ACS Nano 11, 11082–11090 (2017).
Abraham, J. et al. Tunable sieving of ions using graphene oxide membranes. Nat. Nanotechnol. 12, 546–550 (2017).
Liu, H., Wang, H. & Zhang, X. Facile fabrication of freestanding ultrathin reduced graphene oxide membranes for water purification. Adv. Mater. 27, 249–254 (2015).
Chen, L. et al. Ion sieving in graphene oxide membranes via cationic control of interlayer spacing. Nature 550, 380–383 (2017).
Ren, C. E. et al. Voltage-gated ions sieving through 2D MXene Ti3C2Tx membranes. ACS Appl. Nano Mater. 1, 3644–3652 (2018).
Anasori, B., Lukatskaya, M. R. & Gogotsi, Y. 2D metal carbides and nitrides (MXenes) for energy storage. Nat. Rev. Mater. 2, 16098 (2017).
Lukatskaya, M. R. et al. Cation intercalation and high volumetric capacitance of two-dimensional titanium carbide. Science 341, 1502–1505 (2013).
Naguib, M. et al. Two-dimensional nanocrystals produced by exfoliation of Ti3AlC2. Adv. Mater. 23, 4248–4253 (2011).
Ding, L. et al. MXene molecular sieving membranes for highly efficient gas separation. Nat. Commun. 9, 155 (2018).
Shen, J. et al. 2D MXene nanofilms with tunable gas transport channels. Adv. Funct. Mater. 28, 1801511 (2018).
Ren, C. E. et al. Charge- and size-selective ion sieving through Ti3C2Tx MXene membranes. J. Phys. Chem. Lett. 6, 4026–4031 (2015).
Ding, L. et al. A two-dimensional lamellar membrane: MXene nanosheet stacks. Angew. Chem. Int. Ed. Engl. 56, 1825–1829 (2017).
Pandey, R. P. et al. Ultrahigh-flux and fouling-resistant membrane based on layered silver/MXene (Ti3C2Tx) nanosheets. J. Mater. Chem. A 6, 3522–3533 (2018).
Wang, J. et al. A regularly channeled lamellar membrane for unparalleled water and organics permeation. Angew. Chem. Int. Ed. Engl. 57, 6814–6818 (2018).
Rasool, K. et al. Antibacterial activity of Ti3C2Tx MXene. ACS Nano 10, 3674–3684 (2016).
Berdiyorov, G. R. & Mahmoud, K. A. Effect of surface termination on ion intercalation selectivity of bilayer Ti3C2T2 (T = F, O and OH) MXene. Appl. Surf. Sci. 416, 725–730 (2017).
Ernst, K. H., Grman, D., Hauert, R. & Holländer, E. Fluorine‐Induced corrosion of aluminium microchip bond pads: An XPS and AES analysis. Surf. Interface Anal. 21, 691–696 (1994).
Halim, J. et al. X-ray photoelectron spectroscopy of select multi-layered transition metal carbides (MXenes). Appl. Surf. Sci. 362, 406–417 (2016).
Ghidiu, M. et al. Ion-exchange and cation solvation reactions in Ti3C2 MXene. Chem. Mater. 28, 3507–3514 (2016).
Muckley, E. S. et al. Multimodality of structural, electrical, and gravimetric responses of intercalated MXenes to water. ACS Nano 11, 11118–11126 (2017).
Lipatov, A. et al. Effect of synthesis on quality, electronic properties and environmental stability of individual monolayer Ti3C2 MXene flakes. Adv. Electron. Mater. 2, 1600255 (2016).
Alhabeb, M. et al. Guidelines for synthesis and processing of two-dimensional titanium carbide (Ti3C2Tx MXene). Chem. Mater. 29, 7633–7644 (2017).
Richards, L. A., Schäfer, A. I., Richards, B. S. & Corry, B. The importance of dehydration in determining ion transport in narrow pores. Small 8, 1701–1709 (2012).
Sahu, S., Di Ventra, M. & Zwolak, M. Dehydration as a universal mechanism for ion selectivity in graphene and other atomically thin pores. Nano Lett. 17, 4719–4724 (2017).
Segall, M. et al. First-principles simulation: ideas, illustrations and the CASTEP code. J. Phys. Condens. Matter 14, 2717 (2002).
Perdew, J. P. Generalized gradient approximation made simple. Phys. Rev. Lett. 77, 3865 (1996).
Hamann, D. Norm-conserving pseudopotentials. Phys. Rev. Lett. 43, 1494 (1979).
Li, L. et al. Selective gas diffusion in two-dimensional MXene lamellar membranes: insights from molecular dynamics simulations. J. Mater. Chem. A 6, 11734–11742 (2018).
Wang, X. et al. Reversed thermo-switchable molecular sieving membranes composed of two-dimensional metal-organic nanosheets for gas separation. Nat. Commun. 8, 14460 (2017).
Rappé, A. K., Casewit, C. J., Colwell, K., Goddard Iii, W. & Skiff, W. UFF, a full periodic table force field for molecular mechanics and molecular dynamics simulations. J. Am. Chem. Soc. 114, 10024–10035 (1992).
Kadantsev, E. S., Boyd, P. G., Daff, T. D. & Woo, T. K. Fast and accurate electrostatics in metal organic frameworks with a robust charge equilibration parameterization for high-throughput virtual screening of gas adsorption. J. Phys. Chem. Lett. 4, 3056–3061 (2013).
Berendsen, H., Grigera, J. & Straatsma, T. The missing term in effective pair potentials. J. Phys. Chem. 91, 6269–6271 (1987).
Li, P., Song, L. F. & Merz, K. M. Jr Systematic parameterization of monovalent ions employing the nonbonded model. J. Chem. Theory Comput. 11, 1645–1657 (2015).
Hess, B., Bekker, H., Berendsen, H. J. & Fraaije, J. G. LINCS: a linear constraint solver for molecular simulations. J. Comput. Chem. 18, 1463–1472 (1997).
Feller, S. E., Zhang, Y., Pastor, R. W. & Brooks, B. R. Constant pressure molecular dynamics simulation: the Langevin piston method. J. Chem. Phys. 103, 4613–4621 (1995).
Shirts, M. R., Pitera, J. W., Swope, W. C. & Pande, V. S. Extremely precise free energy calculations of amino acid side chain analogs: comparison of common molecular mechanics force fields for proteins. J. Chem. Phys. 119, 5740–5761 (2003).
Darden, T., York, D. & Pedersen, L. Particle mesh Ewald: an N⋅ log (N) method for Ewald sums in large systems. J. Chem. Phys. 98, 10089–10092 (1993).
Hess, B., Kutzner, C., Van Der Spoel, D. & Lindahl, E. GROMACS 4: algorithms for highly efficient, load-balanced, and scalable molecular simulation. J. Chem. Theory Comput. 4, 435–447 (2008).
Berendsen, H. J., van der Spoel, D. & van Drunen, R. GROMACS: a message-passing parallel molecular dynamics implementation. Comput. Phys. Commun. 91, 43–56 (1995).
Source: Resources - nature.com