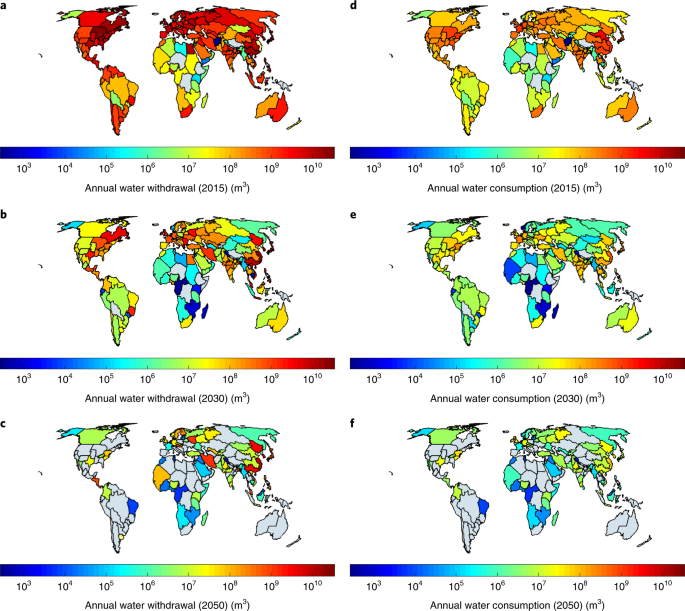
Behrens, P., van Vliet, M. T. H., Nanninga, T., Walsh, B. & Rodrigues, J. F. D. Climate change and the vulnerability of electricity generation to water stress in the European Union. Nat. Energy 2, 17114 (2017).
Ganguli, P., Kumar, D. & Ganguly, A. R. US power production at risk from water stress in a changing climate. Sci. Rep. 7, 11983 (2017).
Water Energy Nexus WEO-2016 Special Report (International Energy Agency, 2016); https://doi.org/10.1021/es903811p
van Vliet, M. T. H. et al. Vulnerability of US and European electricity supply to climate change. Nat. Clim. Change 2, 676–681 (2012).
Roehrkasten, S., Schaeuble, D. & Helgenberger, S. Secure and Sustainable Power Generation in a Water-Constrained World (Institute for Advanced Sustainability Studies, 2015).
McDermott, G. & Nilsen, O. Electricity prices, river temperatures, and cooling water scarcity. Land Econ. 90, 131–148 (2014).
Boogert, A. & Dupont, D. The nature of supply side effects on electricity prices: the impact of water temperature. Econ. Lett. 88, 121–125 (2005).
Spang, E. S., Moomaw, W. R., Gallagher, K. S., Kirshen, P. H. & Marks, D. H. The water consumption of energy production: an international comparison. Environ. Res. Lett. 9, 105002 (2014).
Kenny, J. F. et al. Estimated Use of Water in the United States in 2005 Circular 1344 (US Geological Survey, 2009); https://doi.org/10.3133/cir1405
Luo, T., Krishnaswami, A. & Li, X. A Methodology to Estimate Water Demand for Thermal Power Plants in Data-Scarce Regions using Satellite Images Technical Note (World Research Institute, 2018); https://www.wri.org/publication/methodology-estimate-water-demand-thermal-power-plants-data-scarce-regions
Diehl, T. H., Harris, M. A., Murphy, J. C., Hutson, S. S. & Ladd, D. E. Methods for Estimating Water Consumption for Thermoelectric Power Plants in the United States Scientific Investigations Report 2013–5188 (US Geological Survey, 2013); https://doi.org/10.3133/sir20135188
Flörke, M. et al. Domestic and industrial water uses of the past 60 years as a mirror of socio-economic development: a global simulation study. Glob. Environ. Change 23, 144–156 (2013).
Biesheuvel, A., Witteveen+Bos, Cheng, I., Liu, X. & Greenpeace International. Methods and Results Report: Modelling Global Water Demand for Coal Based Power Generation (Witteveen + Boss, Greenpeace, 2016).
Macknick, J., Newmark, R., Heath, G. & Hallett, K. C. Operational water consumption and withdrawal factors for electricity generating technologies: a review of existing literature. Environ. Res. Lett. 7, 045802 (2012).
van Vliet, M. T. H., Wiberg, D., Leduc, S. & Riahi, K. Power-generation system vulnerability and adaptation to changes in climate and water resources. Nat. Clim. Change 6, 375–380 (2016).
Vassolo, S. & Döll, P. Global-scale gridded estimates of thermoelectric power and manufacturing water use. Water Resour. Res. 41, 1–11 (2005).
Siddiqi, A. & Anadon, L. D. The water–energy nexus in Middle East and North Africa. Energy Policy 39, 4529–4540 (2011).
GWSP Global Water System Project Digital Water Atlas (Global Water System Project, 2008); http://atlas.gwsp.org/
Maulbetsch, J. & Stallings, J. Evaluating the economics of alternative cooling technologies. Power Eng. 116, 120–128 (2012).
Masson-Delmotte et al. Global Warming of 1.5 °C Special Report (IPCC, 2018).
Bogdanov, D. et al. Radical transformation pathway towards sustainable electricity via evolutionary steps. Nat. Commun. 10, 1077 (2019).
Jacobson, M. Z. et al. Matching demand with supply at low cost in 139 countries among 20 world regions with 100% intermittent wind, water, and sunlight (WWS) for all purposes. Renew. Energy 123, 236–248 (2018).
Creutzig, F. et al. The underestimated potential of solar energy to mitigate climate change. Nat. Energy 2, 17140 (2017).
Sgouridis, S., Csala, D. & Bardi, U. The sower’s way: quantifying the narrowing net-energy pathways to a global energy transition. Environ. Res. Lett. 11, 094009 (2016).
Teske, S. Achieving the Paris Climate Agreement Goals (Springer International, 2019).
GlobalData Power (GlobalData Ltd, accessed 20 November 2015); http://power.globaldata.com/Home.aspx
Tracking Clean Energy Progress 2017 (International Energy Agency, 2017); https://www.iea.org/etp/tracking2017/
World Nuclear Performance Report (World Nuclear Association, 2016).
Global Energy Transformation: A Roadmap to 2050 (IRENA, 2019); https://www.irena.org/-/media/Files/IRENA/Agency/Publication/2019/Apr/IRENA_Global_Energy_Transformation_2019.pdf
Hansen, K., Breyer, C. & Lund, H. Status and perspectives on 100% renewable energy systems. Energy 175, 471–480 (2019).
Form EIA-923 Detailed Data with Previous Form Data (EIA-906/920) (US Energy Information Administration, accessed 29 June 2019); https://www.eia.gov/electricity/data/eia923/
Diehl, T. H. & Harris, M. A. Withdrawal and Consumption of Water by Thermoelectric Power Plants in the United States, 2010 Science Investigation Report 2014-5184 (US Geological Survey, 2014); https://doi.org/10.3133/sir20145184
Farfan, J. & Breyer, C. Structural changes of global power generation capacity towards sustainability and the risk of stranded investments supported by a sustainability indicator. J. Clean. Prod. 141, 370–384 (2017).
Wong, C., Williams, C., Pittock, J., Collier, U. & Schelle, P. World’s Top 10 Rivers at Risk (WWF, 2007); http://d2ouvy59p0dg6k.cloudfront.net/downloads/worldstop10riversatriskfinalmarch13_1.pdf
Biggs, E. M. et al. Sustainable development and the water–energy–food nexus: a perspective on livelihoods. Environ. Sci. Policy 54, 389–397 (2015).
Greenpeace, GWEC & SolarPower Europe. Energy [R]evolution: A Sustainable World Energy Outlook 2015 (Greenpeace International, 2015).
Brown, T. W. et al. Response to ‘Burden of proof: A comprehensive review of the feasibility of 100% renewable-electricity systems’. Renew. Sustain. Energy Rev. 92, 834–847 (2018).
Clean Water and Sanitation: Why It Matters (United Nations, 2016); https://www.un.org/sustainabledevelopment/wp-content/uploads/2016/08/6_Why-it-Matters_Sanitation_2p.pdf
World Electric Power Plants Database (S&P Global Platts, 2016).
Renewable Energy Capacity Statistics 2015 (IRENA, 2015).
Lehner, B. et al. High-resolution mapping of the world’s reservoirs and dams for sustainable river-flow management. Front. Ecol. Environ. 9, 494–502 (2011).
Gerlach, A., Werner, C., Gerlach, A., Breyer, C. & Orlandi, S. In Proc. of the 31st European Photovoltaic Solar Energy Conference (eds Rink, S., Helm, P & Taylor, N.) 2965–2973 (WIP Renewable Energies, 2015).
Schaap, D. M. A. & Lowry, R. K. SeaDataNet—Pan-European infrastructure for ocean and marine data management. Int. J. Digit. Earth 3, 50–69 (2010).
Form EIA-860 detailed data with previous form data (EIA-860A/860B) (Energy Information Administration, accessed 29 June 2019); https://www.eia.gov/electricity/data/eia860/
Morton, V. & Echeverri, D. P. Electric Power Plant Water Use in North Carolina: Forced Evaporation and Emission Controls. MSc Thesis, Duke Univ. (2010).
Feeley, T. J. et al. Water: a critical resource in the thermoelectric power industry. Energy 33, 1–11 (2008).
Statistics. Global Energy Data at your Fingertips (International Energy Agency, 2018).
Electric Power Monthly (US Energy Information Administration, accessed 29 June 2019); https://www.eia.gov/electricity/monthly/epm_table_grapher.php?t=epmt_1_01
Wessel, P. & Smith, W. H. F. A global, self-consistent, hierarchical, high-resolution shoreline database. J. Geophys. Res. Solid Earth 101, 8741–8743 (1996).
Zhou, Y. & Tol, R. S. J. Evaluating the costs of desalination and water transport. Water Resour. Res. 41, 1–10 (2005).
Cooling Power Plants (World Nuclear Association, accessed 1 November 2017); http://www.world-nuclear.org/information-library/current-and-future-generation/cooling-power-plants.aspx
Groves, J., Krankkala, T. & Nigent, G. Afton combined cycle with hybrid cooling. Power Engineering 114, 56–60 (2010).
Farfan, J. & Breyer, C. Aging of European power plant infrastructure as an opportunity to evolve towards sustainability. Int. J. Hydrog. Energy 42, 18081–18091 (2017).
Rivers + lake centerlines (Natural Earth, 2016); https://www.naturalearthdata.com/downloads/50m-physical-vectors/50m-rivers-lake-centerlines/
Source: Resources - nature.com