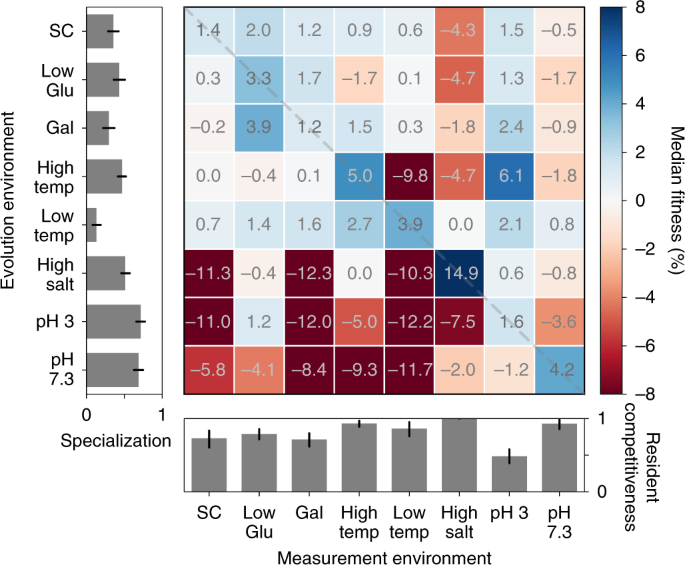
Barrick, J. E. & Lenski, R. E. Genome dynamics during experimental evolution. Nat. Rev. Genet. 14, 827–839 (2013).
Lee, C. E. Evolutionary genetics of invasive species. Trends Ecol. Evol. 17, 386–391 (2002).
Bedhomme, S., Hillung, J. & Elena, S. F. Emerging viruses: why they are not jacks of all trades? Curr. Opin. Virol. 10, 1–6 (2015).
Kassen, R. The experimental evolution of specialists, generalists, and the maintenance of diversity. J. Evol. Biol. 15, 173–190 (2002).
Schluter, D. Evidence for ecological speciation and its alternative. Science 323, 737–741 (2009).
Forister, M., Dyer, L. A., Singer, M., Stireman, J. O. III & Lill, J. Revisiting the evolution of ecological specialization, with emphasis on insect-plant interactions. Ecology 93, 981–991 (2012).
Mitchell-Olds, T., Willis, J. H. & Goldstein, D. B. Which evolutionary processes influence natural genetic variation for phenotypic traits? Nat. Rev. Genet. 8, 845–856 (2007).
Savolainen, O., Lascoux, M. & Merilä, J. Ecological genomics of local adaptation. Nat. Rev. Genet. 14, 807–820 (2013).
Minor, P. D. Live attenuated vaccines: historical successes and current challenges. Virology 479, 379–392 (2015).
Kim, S., Lieberman, T. D. & Kishony, R. Alternating antibiotic treatments constrain evolutionary paths to multidrug resistance. Proc. Natl Acad. Sci. USA 111, 14494–14499 (2014).
Futuyma, D. J. & Moreno, G. The evolution of ecological specialization. Annu. Rev. Ecol. Syst. 19, 207–233 (1988).
Anderson, J. T., Willis, J. H. & Mitchell-Olds, T. Evolutionary genetics of plant adaptation. Trends Genet. 27, 258–266 (2011).
Bono, L. M., Smith, L. B. Jr, Pfennig, D. W. & Burch, C. L. The emergence of performance trade-offs during local adaptation: insights from experimental evolution. Mol. Ecol. 26, 1720–1733 (2017).
Elena, S. F. Local adaptation of plant viruses: lessons from experimental evolution. Mol. Ecol. 26, 1711–1719 (2017).
Levins, R. Evolution in Changing Environments: Some Theoretical Explorations (Princeton Univ. Press, 1968).
MacArthur, R. H. Geographical Ecology: Patterns in the Distribution of Species (Princeton Univ. Press, 1984).
Stearns, S. C. Trade-offs in life-history evolution. Funct. Ecol. 3, 259–268 (1989).
Remold, S. Understanding specialism when the jack of all trades can be the master of all. Proc. Natl Acad. Sci. USA 279, 4861–4869 (2012).
Kawecki, T. J. Accumulation of deleterious mutations and the evolutionary cost of being a generalist. Am. Nat. 144, 833–838 (1994).
Whitlock, M. C. The red queen beats the jack-of-all-trades: the limitations on the evolution of phenotypic plasticity and niche breadth. Am. Nat. 148, S65–S77 (1996).
Bono, L. M., Draghi, J. A. & Turner, P. E. Evolvability costs of niche expansion. Trends Genet. 36, 14–23 (2019).
Anderson, J. T., Lee, C.-R., Rushworth, C. A., Colautti, R. I. & Mitchell-Olds, T. Genetic trade-offs and conditional neutrality contribute to local adaptation. Mol. Ecol. 22, 699–708 (2013).
Ågren, J., Oakley, C. G., McKay, J. K., Lovell, J. T. & Schemske, D. W. Genetic mapping of adaptation reveals fitness tradeoffs in Arabidopsis thaliana. Proc. Natl Acad. Sci. USA 110, 21077–21082 (2013).
Tiffin, P. & Ross-Ibarra, J. Advances and limits of using population genetics to understand local adaptation. Trends Ecol. Evol. 29, 673–680 (2014).
Cooper, V. S. & Lenski, R. E. The population genetics of ecological specialization in evolving Escherichia coli populations. Nature 407, 736–739 (2000).
Turner, P. E. & Elena, S. F. Cost of host radiation in an RNA virus. Genetics 156, 1465–1470 (2000).
Zhong, S., Khodursky, A., Dykhuizen, D. E. & Dean, A. M. Evolutionary genomics of ecological specialization. Proc. Natl Acad. Sci. USA 101, 11719–11724 (2004).
MacLean, R. C., Bell, G. & Rainey, P. B. The evolution of a pleiotropic fitness tradeoff in Pseudomonas fluorescens. Proc. Natl Acad. Sci. USA 101, 8072–8077 (2004).
Ostrowski, E. A., Rozen, D. E. & Lenski, R. E. Pleiotropic effects of beneficial mutations in Escherichia coli. Evolution 59, 2343–2352 (2005).
Duffy, S., Turner, P. E. & Burch, C. L. Pleiotropic costs of niche expansion in the RNA bacteriophage ϕ6. Genetics 172, 751–757 (2006).
Bennett, A. F. & Lenski, R. E. An experimental test of evolutionary trade-offs during temperature adaptation. Proc. Natl Acad. Sci. USA 104, 8649–8654 (2007).
Dettman, J. R., Sirjusingh, C., Kohn, L. M. & Anderson, J. B. Incipient speciation by divergent adaptation and antagonistic epistasis in yeast. Nature 447, 585–588 (2007).
Lee, M.-C., Chou, H.-H. & Marx, C. J. Asymmetric, bimodal trade-offs during adaptation of Methylobacterium to distinct growth substrates. Evolution 63, 2816–2830 (2009).
Wenger, J. W. et al. Hunger artists: yeast adapted to carbon limitation show trade-offs under carbon sufficiency. PLoS Genet. 7, e1002202 (2011).
Jasmin, J.-N., Dillon, M. M. & Zeyl, C. The yield of experimental yeast populations declines during selection. Proc. Natl Acad. Sci. USA 279, 4382–4388 (2012).
Jasmin, J.-N. & Zeyl, C. Evolution of pleiotropic costs in experimental populations. J. Evol. Biol. 26, 1363–1369 (2013).
Yi, X. & Dean, A. M. Bounded population sizes, fluctuating selection and the tempo and mode of coexistence. Proc. Natl Acad. Sci. USA 110, 16945–16950 (2013).
Hietpas, R. T., Bank, C., Jensen, J. D. & Bolon, D. N. Shifting fitness landscapes in response to altered environments. Evolution 67, 3512–3522 (2013).
Hong, K.-K. & Nielsen, J. Adaptively evolved yeast mutants on galactose show trade-offs in carbon utilization on glucose. Metab. Eng. 16, 78–86 (2013).
Rodríguez-Verdugo, A., Carrillo-Cisneros, D., González-González, A., Gaut, B. S. & Bennett, A. F. Different tradeoffs result from alternate genetic adaptations to a common environment. Proc. Natl Acad. Sci. USA 111, 12121–12126 (2014).
Schick, A., Bailey, S. F. & Kassen, R. Evolution of fitness trade-offs in locally adapted populations of Pseudomonas fluorescens. Am. Nat. 186, S48–S59 (2015).
Leiby, N. & Marx, C. J. Metabolic erosion primarily through mutation accumulation, and not tradeoffs, drives limited evolution of substrate specificity in Escherichia coli. PLoS Biol. 12, e1001789 (2014).
McGee, L. W. et al. Payoffs, not tradeoffs, in the adaptation of a virus to ostensibly conflicting selective pressures. PLoS Genet. 10, e1004611 (2014).
Fraebel, D. T. et al. Environment determines evolutionary trajectory in a constrained phenotypic space. eLife 6, e24669 (2017).
Lalić, J., Cuevas, J. M. & Elena, S. F. Effect of host species on the distribution of mutational fitness effects for an RNA virus. PLoS Genet. 7, e1002378 (2011).
Li, C. & Zhang, J. Multi-environment fitness landscapes of a tRNA gene. Nat. Ecol. Evol. 2, 1025–1032 (2018).
Selmecki, A. M. et al. Polyploidy can drive rapid adaptation in yeast. Nature 519, 349–352 (2015).
Gerstein, A. C., Chun, H.-J. E., Grant, A. & Otto, S. P. Genomic convergence toward diploidy in Saccharomyces cerevisiae. PLoS Genet. 2, e145 (2006).
Venkataram, S. et al. Development of a comprehensive genotype-to-fitness map of adaptation-driving mutations in yeast. Cell 166, 1585–1596 (2016).
Voordeckers, K. et al. Adaptation to high ethanol reveals complex evolutionary pathways. PLoS Genet. 11, e1005635 (2015).
Harari, Y., Ram, Y. & Kupiec, M. Frequent ploidy changes in growing yeast cultures. Curr. Genet. 64, 1001–1004 (2018).
Wickner, R. B. Double-stranded and single-stranded RNA viruses of Saccharomyces cerevisiae. Annu. Rev. Microbiol. 46, 347–375 (1992).
Vagnoli, P., Musmanno, R. A., Cresti, S., DiMaggio, T. & Coratza, G. Occurrence of killer yeasts in spontaneous wine fermentations from the tuscany region of italy. Appl. Environ. Microbiol. 59, 4037–4043 (1993).
Schmitt, M. J. & Breinig, F. Yeast viral killer toxins: lethality and self-protection. Nat. Rev. Microbiol. 4, 212–221 (2006).
Greig, D. & Travisano, M. Density-dependent effects on allelopathic interactions in yeast. Evolution 62, 521–527 (2008).
Pieczynska, M. D., de Visser, J. A. G. & Korona, R. Incidence of symbiotic dsRNA killer viruses in wild and domesticated yeast. FEMS Yeast Res. 13, 856–859 (2013).
Kandel, J. S. in Viruses of Fungi and Simple Eukaryotes (eds Koltin, Y. & Leibowitz, M. J.) Ch. 11 (CRC Press, 1988).
Schmitt, M. J. & Breinig, F. The viral killer system in yeast: from molecular biology to application. FEMS Microbiol. Rev. 26, 257–276 (2002).
Buskirk, S. W., Rokes, A. B. & Lang, G. I. Adaptive evolution of a rock-paper-scissors sequence along a direct line of descent. Preprint at bioRxiv https://doi.org/10.1101/700302 (2019).
Liu, H. & Zhang, J. Yeast spontaneous mutation rate and spectrum vary with environment. Curr. Biol. 29, 1584–1591 (2019).
Sexton, J. P., Montiel, J., Shay, J. E., Stephens, M. R. & Slatyer, R. A. Evolution of ecological niche breadth. Annu. Rev. Ecol. Evol. Syst. 48, 183–206 (2017).
Good, B. H., Rouzine, I. M., Balick, D. J., Hallatschek, O. & Desai, M. M. Distribution of fixed beneficial mutations and the rate of adaptation in asexual populations. Proc. Natl Acad. Sci. USA 109, 4950–4955 (2012).
Tikhonov, M., Kachru, S. & Fisher, D. S. Modeling the interplay between plastic tradeoffs and evolution in changing environments. Preprint at bioRxiv https://doi.org/10.1101/711531 (2019).
Lang, G. I. & Murray, A. W. Estimating the per-base-pair mutation rate in the yeast Saccharomyces cerevisiae. Genetics 178, 67–82 (2008).
Lang, G. I., Botstein, D. & Desai, M. M. Genetic variation and the fate of beneficial mutations in asexual populations. Genetics 188, 647–661 (2011).
Kryazhimskiy, S., Rice, D. P., Jerison, E. R. & Desai, M. M. Global epistasis makes adaptation predictable despite sequence-level stochasticity. Science 344, 1519–1522 (2014).
Baym, M. et al. Inexpensive multiplexed library preparation for megabase-sized genomes. PLoS ONE 10, e0128036 (2015).
Jerison, E. R. et al. Genetic variation in adaptability and pleiotropy in budding yeast. eLife 6, e27167 (2017).
Lang, G. I. et al. Pervasive genetic hitchhiking and clonal interference in forty evolving yeast populations. Nature 500, 571–574 (2013).
Sherman, F., Fink, G. R. & Hicks, J. B. Methods in Yeast Genetics: Laboratory Manual (Cold Spring Harbor, 1981).
Storici, F., Lewis, L. K. & Resnick, M. A. In vivo site-directed mutagenesis using oligonucleotides. Nat. Biotechnol. 19, 773–776 (2001).
Source: Ecology - nature.com