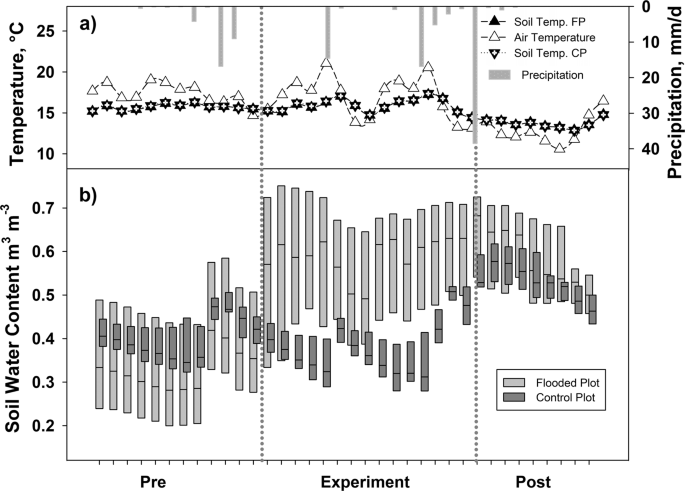
Intergovernmental Panel on Climate Change. Climate Change 2014: Mitigation of Climate Change: Working Group III Contribution to the IPCC Fifth Assessment Report. Working Group III Contribution to the Fifth Assessment Report of the Intergovernmental Panel on Climate Change. https://doi.org/10.1017/CBO9781107415416 (2014).
Saikawa, E. et al. Global and regional emissions estimates for N2O. Atmos. Chem. Phys. 14, 4617–4641 (2014).
Tian, H. et al. The terrestrial biosphere as a net source of greenhouse gases to the atmosphere. Nature 531, 225–228 (2016).
Dalal, R. C. & Allen, D. E. Greenhouse gas fluxes from natural ecosystems. Aust. J. Bot. 56, 369 (2008).
Covey, K. R., University, Y. & Megonigal, J. P. Methane production and emissions in trees and forests. New Phytol. https://doi.org/10.1111/nph.15624 (2018).
Barba, J. et al. Methane emissions from tree stems: a new frontier in the global carbon cycle. New Phytol. https://doi.org/10.1111/nph.15582 (2018).
Megonigal, J. P. & Guenther, A. B. Methane emissions from upland forest soils and vegetation. Tree Physiol. 28, 491–498 (2008).
Gauci, V., Gowing, D. J. G., Hornibrook, E. R. C., Davis, J. M. & Dise, N. B. Woody stem methane emission in mature wetland alder trees. Atmos. Environ. 44, 2157–2160 (2010).
Pangala, S. R., Moore, S., Hornibrook, E. R. C. & Gauci, V. Trees are major conduits for methane egress from tropical forested wetlands. New Phytol. 197, 524–531 (2013).
Smith, Ka. A. et al. Exchange of greenhousegases between soil and atmosphere: interactions of soil physical factors and biological processes. Eur. J. Soil Sci. 54, 779–791 (2003).
Rusch, H. & Rennenberg, H. Black alder (Alnus glutinosa (L.) Gaertn.) trees mediate methane and nitrous oxide emission from the soil to the atmosphere. Plant Soil 201, 1–7 (1998).
Machacova, K., Papen, H., Kreuzwieser, J. & Rennenberg, H. Inundation strongly stimulates nitrous oxide emissions from stems of the upland tree Fagus sylvatica and the riparian tree Alnus glutinosa. Plant and Soil vol. 364 (2013).
Pangala, S. R., Hornibrook, E. R. C., Gowing, D. J. & Gauci, V. The contribution of trees to ecosystem methane emissions in a temperate forested wetland. Glob. Chang. Biol. 21, 2642–2654 (2015).
Terazawa, K., Ishizuka, S., Sakata, T., Yamada, K. & Takahashi, M. Methane emissions from stems of Fraxinus mandshurica var. japonica trees in a floodplain forest. Soil Biol. Biochem. 39, 2689–2692 (2007).
Aosaar, J., Varik, M. & Uri, V. Biomass production potential of grey alder (Alnus incana (L.) Moench.) in Scandinavia and Eastern Europe: A review. Biomass and Bioenergy 45, 11–26 (2012).
Uri, V., Lõhmus, K., Kiviste, A. & Aosaar, J. The dynamics of biomass production in relation to foliar and root traits in a grey alder (Alnus incana (L.) Moench) plantation on abandoned agricultural land. Forestry 82, 61–74 (2009).
Uri, V., Tullus, H. & Lo, K. Uri (2001) Biomass production and nutrien accumulation in short-rotation grey alder.pdf. 161, 169–179 (2002).
Rytter, L. & Rytter, R. M. Growth and carbon capture of grey alder (Alnus incana (L.) Moench.) under north European conditions – Estimates based on reported research. For. Ecol. Manage. 373, 56–65 (2016).
Evans, J. Silviculture of Broadleaved Woodland. J. Appl. Ecol. 22, 610 (2006).
Vogel, C. S., Curtis, P. S. & Thomas, R. B. Growth and nitrogen accretion of dinitrogen-fixing Alnus glutinosa (L.) Gaertn. under elevated carbon dioxide. Plant Ecol. 130, 63–70 (1997).
Krzaklewski, W., Pietrzykowski, M. & WoŚ, B. Survival and growth of alders (Alnus glutinosa (L.) Gaertn. and Alnus incana (L.) Moench) on fly ash technosols at different substrate improvement. Ecol. Eng. 49, 35–40 (2012).
Rosenvald, K. et al. Rhizosphere effect and fine-root morphological adaptations in a chronosequence of silver birch stands on reclaimed oil shale post-mining areas. Ecol. Eng. 37, 1027–1034 (2011).
Šourková, M., Frouz, J. & Šantrůčková, H. Accumulation of carbon, nitrogen and phosphorus during soil formation on alder spoil heaps after brown-coal mining, near Sokolov (Czech Republic). Geoderma 124, 203–214 (2005).
Roy, S., Khasa, D. P. & Greer, C. W. Combining alders, frankiae, and mycorrhizae for the revegetation and remediation of contaminated ecosystems. Can. J. Bot. 85, 237–251 (2007).
Huth, V. et al. The climate warming effect of a fen peat meadow with fluctuating water table is reduced by young alder trees. 21, 1–18 (2018).
Biology, Controls and Models of Tree Volatile Organic Compound Emissions. vol. 5 (Springer Netherlands, (2013).
Maier, M., Machacova, K., Lang, F., Svobodova, K. & Urban, O. Combining soil and tree-stem flux measurements and soil gas profiles to understand CH4 pathways in Fagus sylvatica forests. J. Plant Nutr. Soil Sci. 181, 31–35 (2018).
Machacova, K., Maier, M., Svobodova, K., Lang, F. & Urban, O. Cryptogamic stem covers may contribute to nitrous oxide consumption by mature beech trees. Sci. Rep. 7, 1–7 (2017).
Niinemets, Ü. et al. Environmental feedbacks in temperate aquatic ecosystems under global change: why do we need to consider chemical stressors? Reg. Environ. Chang. 17, 2079–2096 (2017).
Unger, I. M., Kennedy, A. C. & Muzika, R.-M. Flooding effects on soil microbial communities. Appl. Soil Ecol. 42, 1–8 (2009).
Unger, I. M., Motavalli, P. P. & Muzika, R.-M. Changes in soil chemical properties with flooding: A field laboratory approach. Agric. Ecosyst. Environ. 131, 105–110 (2009).
Lohila, A. et al. Responses of N2O fluxes to temperature, water table and N deposition in a northern boreal fen. Eur. J. Soil Sci. 61, 651–661 (2010).
Maljanen, M. et al. The emissions of nitrous oxide and methane from natural soil temperature gradients in a volcanic area in southwest Iceland. Soil Biol. Biochem. 109, 70–80 (2017).
Mer, J. L., Roger, P., Provence, D. & Luminy, D. of methane by soils: A review. Archaea 37, 25–50 (2001).
Butterbach-Bahl, K., Baggs, E. M., Dannenmann, M., Kiese, R. & Zechmeister-Boltenstern, S. Nitrous oxide emissions from soils: How well do we understand the processes and their controls? Philos. Trans. R. Soc. B Biol. Sci. 368 (2013).
Pärn, J. et al. Nitrogen-rich organic soils under warm well-drained conditions are global nitrous oxide emission hotspots. Nat. Commun. 9, 1–8 (2018).
Klemedtsson, L., Svensson, B. H. & Rosswall, T. Relationships between soil moisture content and nitrous oxide production during nitrification and denitrification. Biol. Fertil. Soils 6, 106–111 (1988).
Bateman, E. J. & Baggs, E. M. Contributions of nitrification and denitrification to N2O emissions from soils at different water-filled pore space. Biol. Fertil. Soils 41, 379–388 (2005).
Klemedtsson, L., Svensson, B. H. & Rosswall, T. A method of selective inhibition to distinguish between nitrification and denitrification as sources of nitrous oxide in soil. Biol. Fertil. Soils 6, 112–119 (1988).
Davidson, E. A. & Swank, W. T. Environmental parameters regulating gaseous nitrogen losses from two forested ecosystems via nitrification and denitrification. Appl. Environ. Microbiol. 52, 1287–1292 (1986).
Keppler, F., Hamilton, J. T. G., Braß, M. & Röckmann, T. Methane emissions from terrestrial plants under aerobic conditions. Nature 439, 187–191 (2006).
Terazawa, K., Yamada, K., Ohno, Y., Sakata, T. & Ishizuka, S. Spatial and temporal variability in methane emissions from tree stems of Fraxinus mandshurica in a cool-temperate floodplain forest. Biogeochemistry 123, 349–362 (2015).
Mander, Ü. et al. The impact of a pulsing groundwater table on greenhouse gas emissions in riparian grey alder stands. Environ. Sci. Pollut. Res. 22, 2360–2371 (2015).
Rice, A. L. et al. Emissions of anaerobically produced methane by trees. Geophys. Res. Lett. 37, n/a–n/a (2010).
Pitz, S. L., Megonigal, J. P., Chang, C. H. & Szlavecz, K. Methane fluxes from tree stems and soils along a habitat gradient. Biogeochemistry 137, 307–320 (2018).
Machacova, K. et al. Pinus sylvestris as a missing source of nitrous oxide and methane in boreal forest. Sci. Rep. 6, 1–8 (2016).
Machacova, K., Vainio, E., Urban, O. & Pihlatie, M. Seasonal dynamics of stem N2O exchange follow the physiological activity of boreal trees. Nat. Commun. 10, 1–13 (2019).
Trenberth, K. E. Changes in precipitation with climate change. Clim. Res. 47, 123–138 (2011).
Nicholls, R. J., Hoozemans, F. M. J. & Marchand, M. Increasing flood risk and wetland losses due to global sea-level rise: Regional and global analyses. Glob. Environ. Chang. 9 (1999).
Semmler, T. & Jacob, D. Modeling extreme precipitation events – A climate change simulation for. Europe. Glob. Planet. Change 44, 119–127 (2004).
Blöschl, G. et al. Changing climate both increases and decreases European river floods. Nature 573, 108–111 (2019).
Vargas, R. & Barba. J. Greenhouse Gas Fluxes From Tree Stems. Trends Plant Sci. 24, 296–299 (2019).
Kupper, P. et al. An experimental facility for free air humidity manipulation (FAHM) can alter water flux through deciduous tree canopy. Environ. Exp. Bot. 72, 432–438 (2011).
Soosaar, K. et al. Dynamics of gaseous nitrogen and carbon fluxes in riparian alder forests. Ecol. Eng. 37, 40–53 (2011).
Livingston, G. P. & Hutchinson, G. L. Enclosure-based measurement of trace gas exchange: Applications and sources of error. in Biogenic Trace Gases: Measuring Emissions from Soil and Water (eds. Matson, P. A. & R.C., H.) 14–51 (Ed. Blackwell Publishing: Oxford, Unitel Kingdom (1995).
Collier, S. M., Ruark, M. D., Oates, L. G., Jokela, W. E. & Dell, C. J. Measurement of greenhouse gas flux from agricultural soils using static chambers. J. Vis. Exp. https://doi.org/10.3791/52110 (2014).
Sjögersten, S. et al. Methane emissions from tree stems in neotropical peatlands. New Phytologist, https://doi.org/10.1111/nph.16178 (2019).
Apha, Water Environment Federation & American Water Works Association. Standard Methods for the Examination of Water and Wastewater (Part 1000–3000). Stand. Methods Exam. Water Wastewater 733, doi: ISBN 9780875532356 (1999).
Dray, S., Dufour, A. Ade4: Analysis of Ecological Data. Explor. Euclidean Methods Environ. Sci. 22 (2007).
Tierney, N. et al. Package ‘ naniar’ R topics documented: (2019).
Kowarik, A. & Templ, M. Imputation with the R package VIM. J. Stat. Softw. 74 (2016).
Josse, J. & Husson, F. missMDA: A Package for Handling Missing Values in Multivariate Data Analysis. J. Stat. Softw. 70 (2016).
Oksanen, J. et al. Package ‘ vegan’. 0–291 (2019).
Wang, Y., Naumann, U., Wright, S. T. & Warton, D. I. Mvabund- an R package for model-based analysis of multivariate abundance data. Methods Ecol. Evol. 3, 471–474 (2012).
Bates, D., Mächler, M., Bolker, B. & Walker, S. Fitting Linear Mixed-Effects Models Using lme4. J. Stat. Softw. 67 (2015).
Kuznetsova, A., Brockhoff, P. B. & Christensen, R. H. B. lmerTest Package: Tests in Linear Mixed Effects Models. J. Stat. Softw. 82 (2017).
Harrison, X. A. et al. A brief introduction to mixed effects modelling and multi-model inference in ecology. PeerJ 2018, 1–32 (2018).
Quian, S. S. Environmental and Ecological Statistics with R. (ISBN9781315370262). https://doi.org/10.1201/9781315370262 2016.
Source: Ecology - nature.com