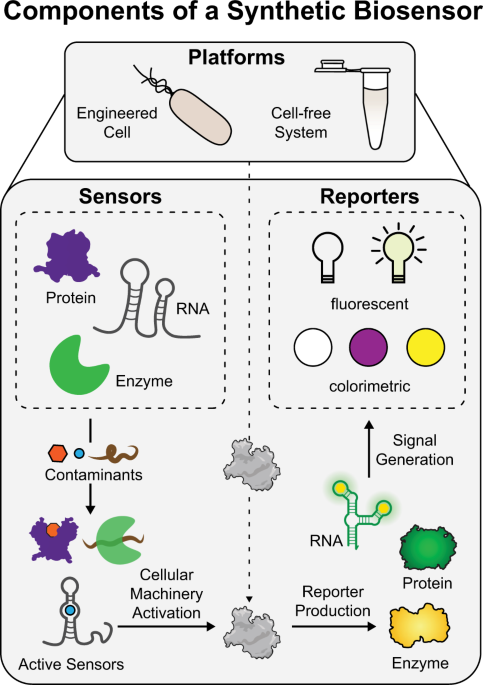
Gosling, S. N. & Arnell, N. W. A global assessment of the impact of climate change on water scarcity. Clim. Change 134, 371–385 (2016).
Kummu, M. et al. The world’s road to water scarcity: shortage and stress in the 20th century and pathways towards sustainability. Sci. Rep. 6, 38495 (2016).
United Nations. High-Level Panel on Water. Making Every Drop Count: An Agenda for Water Action. https://reliefweb.int/report/world/making-every-drop-count-agenda-water-action-high-level-panel-water-outcome-document-14 (2018).
Watkins, K. Human Development Report 2006-beyond Scarcity: Power, Poverty and the Global Water Crisis. (UNDP Human Development Reports, 2006).
Honkonen, T. Water security and climate change: the need for adaptive governance. Potchefstroom Electron. Law Journal/Potchefstroomse Elektron. Regsbl. 20. https://doi.org/10.17159/1727-3781/2016/v19i0a1651 (2017).
World Economic Forum. The Global Risks Report 2019, 14th Edition. https://www.weforum.org/reports/the-global-risks-report-2019 (2019).
USAID. U.S. Government Global Water Strategy. https://www.usaid.gov/what-we-do/water-and-sanitation/us-global-water-strategy (USAID, 2017).
Wagstaff, A. The Millennium Development Goals for Health: Rising to the Challenges. http://documents.worldbank.org/curated/en/875031468329973611/The-millennium-development-goals-for-health-rising-to-the-challenges (2004).
Sachs, J. D. From millennium development goals to sustainable development goals. Lancet 379, 2206–2211 (2012).
World Health Organization & UNICEF. Water for Life: Making It Happen. https://www.who.int/water_sanitation_health/monitoring/jmp2005/en/ (2005).
Bartram, J. et al. Global monitoring of water supply and sanitation: history, methods and future challenges. Int. J. Environ. Res. Public Health 11, 8137–8165 (2014).
Damania, R., Desbureaux, S., Rodella, A.-S., Russ, J. & Zaveri, E. Quality Unknown: The Invisible Water Crisis. (World Bank Publications, 2019).
Stauber, C. The sustainable development goals for water: the need to consider perception, preference, and safety. Am. J. Trop. Med. Hyg. 97, 985 (2017).
UNICEF. SDG Global Indicators Related to Children. https://data.unicef.org/resources/sdg-global-indicators-related-to-children/ (2018).
Ellis, K. et al. Understanding the costs of investigating coliform and E. coli detections during routine drinking water quality monitoring. Urban Water J. 15, 101–108 (2018).
World Health Organization. Guidelines for Drinking-water Quality, 4th edn, Incorporating the 1st Addendum. (World Health Organization, 2018).
Fawell, J., Bailey, K., Chilton, J., Dahi, E. & Magara, Y. Fluoride in Drinking-Water. (IWA Publishing, 2006).
Chelsea Technologies. UviLux. Available at: https://chelsea.co.uk/products/uvilux/.
IDEXX. Coliform/E.coli Water Tests. Available at: https://www.idexx.com/en/water/products/?cy=y_category_252&cx=x_category_259&ts=all.
Hach USA. Arsenic Low Range Test Kit. Available at: https://www.hach.com/arsenic-low-range-test-kit/product?id=7640217303.
Khalil, A. S. & Collins, J. J. Synthetic biology: applications come of age. Nat. Rev. Genet. 11, 367 (2010).
Liu, X. et al. Selective cadmium regulation mediated by a cooperative binding mechanism in CadR. Proc. Natl Acad. Sci. 116, 20398–20403 (2019).
Lucks, J. B., Qi, L., Whitaker, W. R. & Arkin, A. P. Toward scalable parts families for predictable design of biological circuits. Curr. Opin. Microbiol. 11, 567–573 (2008).
Salehi, A. S. M. et al. Cell-free protein synthesis approach to biosensing hTRβ-specific endocrine disruptors. Anal. Chem. 89, 3395–3401 (2017).
Alam, K. K. et al. Rapid, low-cost detection of water contaminants using regulated in vitro transcription. Preprint at biorxiv.org/content/10.1101/619296v2 (2019).
Willardson, B. M. et al. Development and testing of a bacterial biosensor for toluene-based environmental contaminants. Appl. Environ. Microbiol. 64, 1006–1012 (1998).
Tauriainen, S., Karp, M., Chang, W. & Virta, M. Luminescent bacterial sensor for cadmium and lead. Biosens. Bioelectron. 13, 931–938 (1998).
Thavarajah, W. et al. Point-of-use detection of environmental fluoride via a cell-free riboswitch-based biosensor. ACS Synth. Biol. 9, 10–18 (2019).
Selifonova, O., Burlage, R. & Barkay, T. Bioluminescent sensors for detection of bioavailable Hg (II) in the environment. Appl. Environ. Microbiol. 59, 3083–3090 (1993).
Gräwe, A. et al. A paper-based, cell-free biosensor system for the detection of heavy metals and date rape drugs. PLoS ONE 14, e0210940 (2019).
Pellinen, T., Huovinen, T. & Karp, M. A cell-free biosensor for the detection of transcriptional inducers using firefly luciferase as a reporter. Anal. Biochem. 330, 52–57 (2004).
Rawson, D. M., Willmer, A. J. & Turner, A. P. P. Whole-cell biosensors for environmental monitoring. Biosensors 4, 299–311 (1989).
Stocker, J. et al. Development of a set of simple bacterial biosensors for quantitative and rapid measurements of arsenite and arsenate in potable water. Environ. Sci. Technol. 37, 4743–4750 (2003).
Prest, A. G., Winson, M. K., Hammond, J. R. M. & Stewart, G. The construction and application of a lux‐based nitrate biosensor. Lett. Appl. Microbiol. 24, 355–360 (1997).
Virta, M., Lampinen, J. & Karp, M. A luminescence-based mercury biosensor. Anal. Chem. 67, 667–669 (1995).
Wen, K. Y. et al. A cell-free biosensor for detecting quorum sensing molecules in P. aeruginosa-infected respiratory samples. ACS Synth. Biol. 6, 2293–2301 (2017).
Takahashi, M. K. et al. A low-cost paper-based synthetic biology platform for analyzing gut microbiota and host biomarkers. Nat. Commun. 9, 3347 (2018).
Phillips, E. A., Moehling, T. J., Bhadra, S., Ellington, A. D. & Linnes, J. C. Strand displacement probes combined with isothermal nucleic acid amplification for instrument-free detection from complex samples. Anal. Chem. 90, 6580–6586 (2018).
Kawaguchi, T., Chen, Y. P., Norman, R. S. & Decho, A. W. Rapid screening of quorum-sensing signal N-acyl homoserine lactones by an in vitro cell-free assay. Appl. Environ. Microbiol. 74, 3667–3671 (2008).
Jiang, Y. S. et al. Portable platform for rapid in-field identification of human fecal pollution in water. Water Res. 131, 186–195 (2018).
Gootenberg, J. S. et al. Nucleic acid detection with CRISPR-Cas13a/C2c2. Science (80-.). 356, 438–442 (2017).
Pardee, K. et al. Paper-based synthetic gene networks. Cell 159, 940–954 (2014).
Pardee, K. et al. Rapid, low-cost detection of Zika virus using programmable biomolecular components. Cell 165, 1255–1266 (2016).
Verosloff, M., Chappell, J., Perry, K. L., Thompson, J. R. & Lucks, J. B. PLANT-Dx: a molecular diagnostic for point-of-use detection of plant pathogens. ACS Synth. Biol. 8, 902–905 (2019).
Ma, D., Shen, L., Wu, K., Diehnelt, C. W. & Green, A. A. Low-cost detection of norovirus using paper-based cell-free systems and synbody-based viral enrichment. Synth. Biol. 3, ysy018 (2018).
Myhrvold, C. et al. Field-deployable viral diagnostics using CRISPR-Cas13. Science (80-.). 360, 444–448 (2018).
Spoelstra, W. K. et al. CRISPR-based DNA and RNA detection with liquid-liquid phase separation. Preprint at biorxiv.org/content/10.1101/471482v2 (2019).
Romero, J. L. R., Carver, G. D., Johnson, P. A., Perry, K. L. & Thompson, J. R. A rapid, sensitive and inexpensive method for detection of grapevine red blotch virus without tissue extraction using loop-mediated isothermal amplification. Arch. Virol. 164, 1453–1457 (2019).
Grieshaber, D., MacKenzie, R., Vörös, J. & Reimhult, E. Electrochemical biosensors-sensor principles and architectures. Sensors 8, 1400–1458 (2008).
Wang, J. et al. DNA electrochemical biosensors for environmental monitoring. A review. Anal. Chim. Acta 347, 1–8 (1997).
Tyagi, A. et al. Synthetic biology: applications in the food sector. Crit. Rev. Food Sci. Nutr. 56, 1777–1789 (2016).
Mortimer, J. C. Plant synthetic biology could drive a revolution in biofuels and medicine. Exp. Biol. Med. 244, 323–331 (2019).
Slomovic, S., Pardee, K. & Collins, J. J. Synthetic biology devices for in vitro and in vivo diagnostics. Proc. Natl Acad. Sci. 112, 14429–14435 (2015).
Rogers, J. K. et al. Synthetic biosensors for precise gene control and real-time monitoring of metabolites. Nucleic Acids Res. 43, 7648–7660 (2015).
Porto, T. V., Wilson, M. T. & Worrall, J. A. R. Copper and nickel bind via two distinct kinetic mechanisms to a CsoR metalloregulator. Dalt. Trans. 44, 20176–20185 (2015).
Yuan, L., Kurek, I., English, J. & Keenan, R. Laboratory-directed protein evolution. Microbiol. Mol. Biol. Rev. 69, 373–392 (2005).
Porter, E. B., Polaski, J. T., Morck, M. M. & Batey, R. T. Recurrent RNA motifs as scaffolds for genetically encodable small-molecule biosensors. Nat. Chem. Biol. 13, 295 (2017).
Naylor, L. H. Reporter gene technology: the future looks bright. Biochem. Pharmacol. 58, 749–757 (1999).
Thorne, N., Inglese, J. & Auld, D. S. Illuminating insights into firefly luciferase and other bioluminescent reporters used in chemical biology. Chem. Biol. 17, 646–657 (2010).
Wan, X., Ho, T. Y. H. & Wang, B. in Handbook of Cell Biosensors. 1–37 (2019).
Belkin, S. et al. Remote detection of buried landmines using a bacterial sensor. Nat. Biotechnol. 35, 308 (2017).
Stirling, F. et al. Rational design of evolutionarily stable microbial kill switches. Mol. Cell 68, 686–697 (2017).
Silverman, A., Kelley-Loughnane, N., Lucks, J. B. & Jewett, M. C. Deconstructing cell-free extract preparation for in vitro activation of transcriptional genetic circuitry. ACS Synth. Biol. 8, 403–414 (2018).
Pandey, P. K., Kass, P. H., Soupir, M. L., Biswas, S. & Singh, V. P. Contamination of water resources by pathogenic bacteria. AMB Express 4, 51 (2014).
Jamison, D. T. et al. Disease Control Priorities, (Volume 9): Improving Health and Reducing Poverty. (World Bank Publications, 2017).
Pillai, J., Mathew, K., Gibbs, R. & Ho, G. E. H2S paper strip method—A bacteriological test for faecal coliforms in drinking water at various temperatures. Water Sci. Technol. 40, 85–90 (1999).
Aquagenx. The Compartment Bag Test (CBT) Quantitative H2S Water Quality Test Kits. Available at: https://www.aquagenx.com/cbt-h2s/.
Cowburn, J. K., Goodall, T., Fricker, E. J., Walter, K. S. & Fricker, C. R. A preliminary study of the use of Colilert for water quality monitoring. Lett. Appl. Microbiol. 19, 50–52 (1994).
mWater. mWater Test Kits. Available at: https://www.mwater.co/kits.html.
Pall Corporation. Membrane Filter Technique. Available at: https://laboratory.pall.com/en/microbiology-qc/laboratory-membrane-filter-technique.html.
Sorensen, J. P. R. et al. In-situ tryptophan-like fluorescence: a real-time indicator of faecal contamination in drinking water supplies. Water Res. 81, 38–46 (2015).
Zhao, Y., Chen, F., Li, Q., Wang, L. & Fan, C. Isothermal amplification of nucleic acids. Chem. Rev. 115, 12491–12545 (2015).
Kim, Y., Sohn, D. & Tan, W. Molecular beacons in biomedical detection and clinical diagnosis. Int. J. Clin. Exp. Pathol. 1, 105 (2008).
Chen, J. S. et al. CRISPR-Cas12a target binding unleashes indiscriminate single-stranded DNase activity. Science (80-.). 360, 436–439 (2018).
Gootenberg, J. S. et al. Multiplexed and portable nucleic acid detection platform with Cas13, Cas12a, and Csm6. Science (80-.). 360, 439–444 (2018).
Eichmiller, J. J., Best, S. E. & Sorensen, P. W. Effects of temperature and trophic state on degradation of environmental DNA in lake water. Environ. Sci. Technol. 50, 1859–1867 (2016).
Nickson, R. et al. Arsenic poisoning of Bangladesh groundwater. Nature 395, 338 (1998).
Hughes, M. F., Beck, B. D., Chen, Y., Lewis, A. S. & Thomas, D. J. Arsenic exposure and toxicology: a historical perspective. Toxicol. Sci. 123, 305–332 (2011).
Kaur, H., Kumar, R., Babu, J. N. & Mittal, S. Advances in arsenic biosensor development–a comprehensive review. Biosens. Bioelectron. 63, 533–545 (2015).
Brindha, K. & Elango, L. Fluoride in groundwater: causes, implications and mitigation measures. Fluoride Prop. Appl. Environ. Manag. 1, 111–136 (2011).
Hanna Instruments. Fluoride Portable Meter. Available at: https://www.hannainst.com/fluoride-portable-meter-hi98402.html.
Didovyk, A., Tonooka, T., Tsimring, L. & Hasty, J. Rapid and scalable preparation of bacterial lysates for cell-free gene expression. ACS Synth. Biol. 6, 2198–2208 (2017).
McNerney, M. P. et al. Point-of-care biomarker quantification enabled by sample-specific calibration. Sci. Adv. 5, eaax4473 (2019).
Hua, A., Gueuné, H., Cregut, M., Thouand, G. & Durand, M. -J. Development of a bacterial bioassay for atrazine and cyanuric acid detection. Front. Microbiol. 6, 211 (2015).
Silverman, A. D., Akova, U., Alam, K. K., Jewett, M. C. & Lucks, J. B. Design and optimization of a cell-free atrazine biosensor. ACS Synth. Biol. https://doi.org/10.1021/acssynbio.9b00388 (2020).
Karig, D. K., Iyer, S., Simpson, M. L. & Doktycz, M. J. Expression optimization and synthetic gene networks in cell-free systems. Nucleic Acids Res. 40, 3763–3774 (2011).
Jepson, W. E., Wutich, A., Colllins, S. M., Boateng, G. O. & Young, S. L. Progress in household water insecurity metrics: a cross‐disciplinary approach. Wiley Interdiscip. Rev. Water 4, e1214 (2017).
Young, S. L. et al. The Household Water InSecurity Experiences (HWISE) Scale: development and validation of a household water insecurity measure for low-income and middle-income countries. BMJ Glob. Heal. 4, e001750 (2019).
Wutich, A. et al. Advancing methods for research on household water insecurity: studying entitlements and capabilities, socio-cultural dynamics, and political processes, institutions and governance. Water Secur. 2, 1–10 (2017).
Silverman, A. D., Karim, A. S. & Jewett, M. C. Cell-free gene expression: an expanded repertoire of applications. Nat. Rev. Genet. 21, 151–170 (2020).
Huang, P. -S., Boyken, S. E. & Baker, D. The coming of age of de novo protein design. Nature 537, 320 (2016).
Lee, Y. J. & Moon, T. S. Design rules of synthetic non-coding RNAs in bacteria. Methods 143, 58–69 (2018).
Nandagopal, N. & Elowitz, M. B. Synthetic biology: integrated gene circuits. Science (80-.). 333, 1244–1248 (2011).
Alberts, B. et al. Molecular Biology of the Cell 6th edn. (Garland Science, 2015).
Source: Resources - nature.com