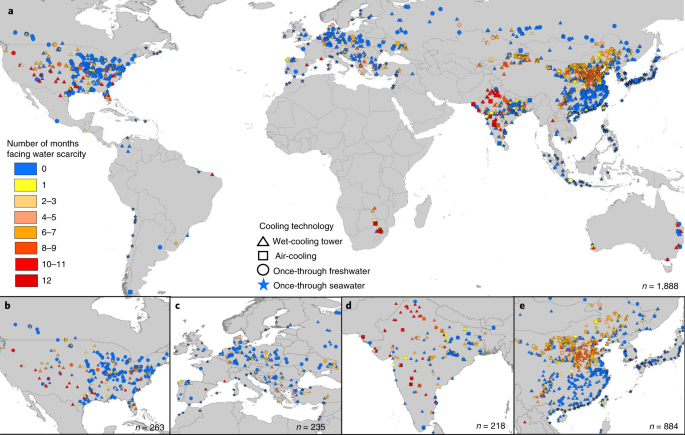
Statistical Review of World Energy 2018 (BP, 2018).
Tong, D. et al. Targeted emission reductions from global super-polluting power plant units. Nat. Sustain. 1, 59–68 (2018).
Oberschelp, C., Pfister, S., Raptis, C. E. & Hellweg, S. Global emission hotspots of coal power generation. Nat. Sustain. 2, 113–121 (2019).
Paris Agreement (European Commission, 2015); https://go.nature.com/3auVx0C
Davis, S. J. & Socolow, R. H. Commitment accounting of CO2 emissions. Environ. Res. Lett. 9, 084018 (2014).
Pfeiffer, A., Hepburn, C., Vogt-Schilb, A. & Caldecott, B. Committed emissions from existing and planned power plants and asset stranding required to meet the Paris Agreement. Environ. Res. Lett. 13, 054019 (2018).
Voisin, N. et al. Vulnerability of the US western electric grid to hydro-climatological conditions: how bad can it get? Energy 115, 1–12 (2016).
Webster, M., Donohoo, P. & Palmintier, B. Water–CO2 trade-offs in electricity generation planning. Nat. Clim. Change 3, 1029–1032 (2013).
Kyle, P. et al. Influence of climate change mitigation technology on global demands of water for electricity generation. Int. J. Greenhouse Gas Control 13, 112–123 (2013).
Byers, E. A., Hall, J. W. & Amezaga, J. M. Electricity generation and cooling water use: UK pathways to 2050. Glob. Environ. Change 25, 16–30 (2014).
Liu, L. et al. Water demands for electricity generation in the US: modeling different scenarios for the water–energy nexus. Technol. Forecast. Soc. Change 94, 318–334 (2015).
Van Vliet, M. T., Wiberg, D., Leduc, S. & Riahi, K. Power-generation system vulnerability and adaptation to changes in climate and water resources. Nat. Clim. Change 6, 375–380 (2016).
World Energy Outlook 2016 (International Energy Agency, 2016).
Zhang, X. et al. China’s coal-fired power plants impose pressure on water resources. J. Clean. Prod. 161, 1171–1179 (2017).
Miara, A. et al. Climate and water resource change impacts and adaptation potential for US power supply. Nat. Clim. Change 7, 793–798 (2017).
Zhang, C., Zhong, L. & Wang, J. Decoupling between water use and thermoelectric power generation growth in China. Nat. Energy 3, 792–799 (2018).
Van Vliet, M. T. et al. Vulnerability of US and European electricity supply to climate change. Nat. Clim. Change 2, 676–681 (2012).
Turner, S. W. D., Voisin, N., Fazio, J., Hua, D. & Jourabchi, M. Compound climate events transform electrical power shortfall risk in the Pacific Northwest. Nat. Commun. 10, 8 (2019).
Liu, L., Hejazi, M., Iyer, G. & Forman, B. A. Implications of water constraints on electricity capacity expansion in the United States. Nat. Sustain. 2, 206–213 (2019).
Alkon, M. et al. Water security implications of coal-fired power plants financed through China’s Belt and Road Initiative. Energy Policy 132, 1101–1109 (2019).
Wang, Y. et al. Vulnerability of existing and planned coal-fired power plants in developing Asia to changes in climate and water resources. Energy Environ. Sci. 12, 3164–3181 (2019).
Rosa, L., Chiarelli, D. D., Rulli, M. C., Dell’Angelo, J. & D’Odorico, P. Global agricultural economic water scarcity. Sci. Adv. 6, eaaz6031 (2020).
D’Odorico, P. et al. The global food–energy–water nexus. Rev. Geophys. 56, 456–531 (2018).
Tong, D. et al. Committed emissions from existing energy infrastructure jeopardize 1.5 °C climate target. Nature 572, 373–377 (2019).
Cui, R. Y. et al. Quantifying operational lifetimes for coal power plants under the Paris goals. Nat. Commun. 10, 4759 (2019).
Smit, B., Reimer, J. A., Oldenburg, C. M. & Bourg, I. C. Introduction to Carbon Capture and Sequestration (Imperial College Press, 2014).
Bui, M. et al. Carbon capture and storage (CCS): the way forward. Energy Environ. Sci. 11, 1062–1176 (2018).
Zhai, H. & Rubin, E. S. Water impacts of a low-carbon electric power future: assessment methodology and status. Curr. Sustain. Renew. Energy Rep. 2, 1–9 (2015).
Zhai, H. & Rubin, E. S. Performance and cost of wet and dry cooling systems for pulverized coal power plants with and without carbon capture and storage. Energy Policy 38, 5653–5660 (2010).
Meldrum, J., Nettles-Anderson, S., Heath, G. & Macknick, J. Life cycle water use for electricity generation: a review and harmonization of literature estimates. Environ. Res. Lett. 8, 015031 (2013).
Zhai, H., Rubin, E. S. & Versteeg, P. L. Water use at pulverized coal power plants with post-combustion carbon capture and storage. Environ. Sci. Technol. 45, 2479–2485 (2011).
Tidwell, V. C., Malczynski, L. A., Kobos, P. H., Klise, G. T. & Shuster, E. Potential impacts of electric power production utilizing natural gas, renewables and carbon capture and sequestration on US freshwater resources. Environ. Sci. Technol. 47, 8940–8947 (2013).
Talati, S., Zhai, H. & Morgan, M. G. Water impacts of CO2 emission performance standards for fossil fuel-fired power plants. Environ. Sci. Technol. 48, 11769–11776 (2014).
Sathre, R. et al. Spatially-explicit water balance implications of carbon capture and sequestration. Environ. Model. Softw. 75, 153–162 (2016).
Eldardiry, H. & Habib, E. Carbon capture and sequestration in power generation: review of impacts and opportunities for water sustainability. Energy Sustain. Soc. 8, 6 (2018).
Schakel, W., Pfister, S. & Ramírez, A. Exploring the potential impact of implementing carbon capture technologies in fossil fuel power plants on regional European water stress index levels. Int. J. Greenhouse Gas Control 39, 318–328 (2015).
Byers, E. A., Hall, J. W., Amezaga, J. M., O’Donnell, G. M. & Leathard, A. Water and climate risks to power generation with carbon capture and storage. Environ. Res. Lett. 11, 024011 (2016).
Integrated Environmental Control Model computer code and documentation (IECM, 2009); https://www.cmu.edu/epp/iecm/iecm_dl.html
Rogelj, J. et al. in Special Report on Global Warming of 1.5 °C (eds Masson-Delmotte, V. et al.) Ch. 2 (IPCC, WMO, 2018).
Rochelle, G. T. Amine scrubbing for CO2 capture. Science 325, 1652–1654 (2009).
Flörke, M., Schneider, C. & McDonald, R. I. Water competition between cities and agriculture driven by climate change and urban growth. Nat. Sustain. 1, 51–58 (2018).
Rosa, L., Rulli, M. C., Davis, K. F. & D’Odorico, P. The water–energy nexus of hydraulic fracturing: a global hydrologic analysis for shale oil and gas extraction. Earth’s Future 6, 745–756 (2018).
Rosa, L. et al. Closing the yield gap while ensuring water sustainability. Environ. Res. Lett. 13, 104002 (2018).
Rosa, L., Chiarelli, D. D., Tu, C., Rulli, M. C. & D’Odorico, P. Global unsustainable virtual water flows in agricultural trade. Environ. Res. Lett. 14, 114001 (2019).
World Energy Outlook 2015 (International Energy Agency, 2015).
Powell, S., Liu, K., Liu, A., Li, W. & Hudson, J. Is China Consuming too Much Water to Make Electricity? (UBS Evidence Lab, 2016); https://neo.ubs.com/shared/d1k4sjYSwbRh/
Pastor, A. V., Ludwig, F., Biemans, H., Hoff, H. & Kabat, P. Accounting for environmental flow requirements in global water assessments. Hydrol. Earth Syst. Sci. 18, 5041–5059 (2014).
Global Coal Plant Tracker (Global Energy Monitor, accessed 18 April 2020); https://endcoal.org/global-coal-plant-tracker/
Lohrmann, A., Farfan, J., Caldera, U., Lohrmann, C. & Breyer, C. Global scenarios for significant water use reduction in thermal power plants based on cooling water demand estimation using satellite imagery. Nat. Energy 4, 1040–1048 (2019).
Fick, S. E. & Hijmans, R. J. WorldClim 2: new 1‐km spatial resolution climate surfaces for global land areas. Int. J. Climatol. 37, 4302–4315 (2017).
Grubert, E. A., Beach, F. C. & Webber, M. E. Can switching fuels save water? A life cycle quantification of freshwater consumption for Texas coal- and natural gas-fired electricity. Environ. Res. Lett. 7, 045801 (2012).
Jordaan, S. M., Patterson, L. A. & Anadon, L. D. A spatially-resolved inventory analysis of the water consumed by the coal-to-gas transition of Pennsylvania. J. Clean. Prod. 184, 366–374 (2018).
Rosa, L. & D’Odorico, P. The water–energy–food nexus of unconventional oil and gas extraction in the Vaca Muerta Play, Argentina. J. Clean. Prod. 207, 743–750 (2019).
Sutanudjaja, E. H. et al. PCR-GLOBWB 2: a 5 arcmin global hydrological and water resources model. Geosci. Model Dev. 11, 2429–2453 (2018).
Wanders, N., van Vliet, M. T., Wada, Y., Bierkens, M. F. & van Beek, L. P. High‐resolution global water temperature modeling. Water Resour. Res. 55, 2760–2778 (2019).
Hoekstra, A. Y. & Mekonnen, M. M. The water footprint of humanity. Proc. Natl Acad. Sci. USA 109, 3232–3237 (2012).
Richter, B. D., Davis, M. M., Apse, C. & Konrad, C. A presumptive standard for environmental flow protection. River Res. Appl. 28, 1312–1321 (2012).
Davidson, C. L., Dooley, J. J. & Dahowski, R. T. Assessing the impacts of future demand for saline groundwater on commercial deployment of CCS in the United States. Energy Procedia 1, 1949–1956 (2009).
Little, M.G. & Jackson, R. B. Potential impacts of leakage from deep CO2 geosequestration on overlying freshwater aquifers. Environ. Sci. Technol. 44, 9225–9232 (2010).
Zhang, C., Anadon, L. D., Mo, H., Zhao, Z. & Liu, Z. Water–carbon trade-off in China’s coal power industry. Environ. Sci. Technol. 48, 11082–11089 (2014).
Peer, R. A. & Sanders, K. T. The water consequences of a transitioning US power sector. Appl. Energy 210, 613–622 (2018).
Macknick, J., Newmark, R., Heath, G. & Hallett, K. C. Operational water consumption and withdrawal factors for electricity generating technologies: a review of existing literature. Environ. Res. Lett. 7, 045802 (2012).
Scanlon, B. R., Duncan, I. & Reedy, R. C. Drought and the water–energy nexus in Texas. Environ. Res. Lett. 8, 045033 (2013).
Siegelman, R. L., Milner, P. J., Kim, E. J., Weston, S. C. & Long, J. R. Challenges and opportunities for adsorption-based CO2 capture from natural gas combined cycle emissions. Energy Environ. Sci. 12, 2161–2173 (2019).
Davis, S. J. et al. Net-zero emissions energy systems. Science 360, eaas9793 (2018).
Kätelhön, A., Meys, R., Deutz, S., Suh, S. & Bardow, A. Climate change mitigation potential of carbon capture and utilization in the chemical industry. Proc. Natl Acad. Sci. USA 116, 11187–11194 (2019).
Boot-Handford, M. E. et al. Carbon capture and storage update. Energy Environ. Sci. 7, 130–189 (2014).
Sanchez, D. L., Nelson, J. H., Johnston, J., Mileva, A. & Kammen, D. M. Biomass enables the transition to a carbon-negative power system across western North America. Nat. Clim. Change 5, 230–234 (2015).
Realmonte, G. et al. An inter-model assessment of the role of direct air capture in deep mitigation pathways. Nat. Commun. 10, 3277 (2019).
Source: Resources - nature.com