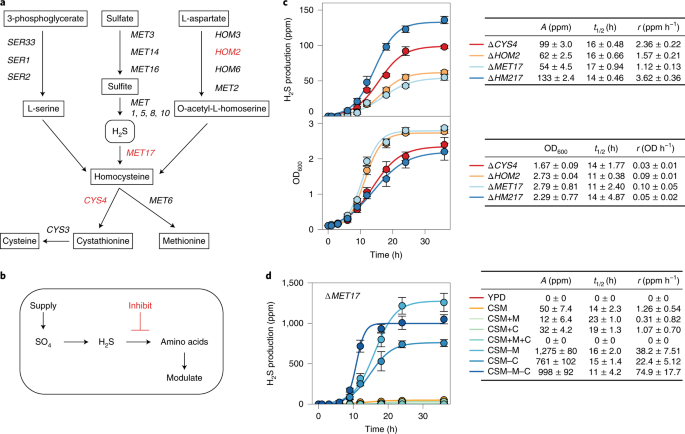
Rucevska, I. et al. Waste Crime–Waste Risks: Gaps in Meeting the Global Waste Challenge (UNEP and GRID-Arendal, 2015); https://go.nature.com/38RZ1cY
Balde, C. P., Forti, V., Gray, V., Kuehr, R. & Stegmann, P. The Global e-Waste Monitor 2017: Quantities, Flows and Resources (United Nations University, International Telecommunication Union and International Solid Waste Association, 2017).
Statistics: All Mining (CDC, 2018); https://www.cdc.gov/niosh/mining/statistics/allmining.html
DeGraff, J. V. in Understanding and Responding to Hazardous Substances at Mine Sites in the Western United States (ed. DeGraff, J. V.) 1–8 (Geological Society of America, 2007).
Fu, F. & Wang, Q. Removal of heavy metal ions from wastewaters: a review. J. Environ. Manage. 92, 407–418 (2011).
Kang, D. H. P., Chen, M. & Ogunseitan, O. A. Potential environmental and human health impacts of rechargeable lithium batteries in electronic waste. Environ. Sci. Technol. 47, 5495–5503 (2013).
Song, X., Hu, S., Chen, D. & Zhu, B. Estimation of waste battery generation and analysis of the waste battery recycling system in China. J. Ind. Ecol. 21, 57–69 (2017).
Kurniawan, T. A., Chan, G. Y. S., Lo, W.-H. & Babel, S. Physico–chemical treatment techniques for wastewater laden with heavy metals. Chem. Eng. J. 118, 83–98 (2006).
Barakat, M. A. New trends in removing heavy metals from industrial wastewater. Arab. J. Chem. 4, 361–377 (2011).
Gupta, K. V., Ali, I., A. Saleh, T., Nayak, A. & Agarwal, S. Chemical treatment technologies for waste-water recycling—an overview. RSC Adv. 2, 6380–6388 (2012).
Gavrilescu, M., Demnerová, K., Aamand, J., Agathos, S. & Fava, F. Emerging pollutants in the environment: present and future challenges in biomonitoring, ecological risks and bioremediation. New Biotechnol. 32, 147–156 (2015).
Singh, A. & Prasad, S. M. Remediation of heavy metal contaminated ecosystem: an overview on technology advancement. Int. J. Environ. Sci. Technol. 12, 353–366 (2015).
Gadd, G. M. Microbial influence on metal mobility and application for bioremediation. Geoderma 122, 109–119 (2004).
Wiatrowski, H. A., Ward, P. M. & Barkay, T. Novel reduction of mercury (II) by mercury-sensitive dissimilatory metal reducing bacteria. Environ. Sci. Technol. 40, 6690–6696 (2006).
Silver, S. & Phung, L. T. Genes and enzymes involved in bacterial oxidation and reduction of inorganic arsenic. Appl. Environ. Microbiol. 71, 599–608 (2005).
Picard, A., Gartman, A., Clarke, D. R. & Girguis, P. R. Sulfate-reducing bacteria influence the nucleation and growth of mackinawite and greigite. Geochim. Cosmochim. Acta 220, 367–384 (2018).
Gartman, A. et al. Microbes facilitate mineral deposition in bioelectrochemical systems. ACS Earth Space Chem. 1, 277–287 (2017).
Jong, T. & Parry, D. L. Removal of sulfate and heavy metals by sulfate reducing bacteria in short-term bench scale upflow anaerobic packed bed reactor runs. Water Res. 37, 3379–3389 (2003).
Kieu, H. T. Q., Müller, E. & Horn, H. Heavy metal removal in anaerobic semi-continuous stirred tank reactors by a consortium of sulfate-reducing bacteria. Water Res. 45, 3863–3870 (2011).
Neculita, C.-M., Zagury, G. J. & Bussière, B. Passive treatment of acid mine drainage in bioreactors using sulfate-reducing bacteria. J. Environ. Qual. 36, 1–16 (2007).
Lovley, D. R. Cleaning up with genomics: applying molecular biology to bioremediation. Nat. Rev. Microbiol. 1, 35–44 (2003).
Shiratori, T., Inoue, C., Sugawara, K., Kusano, T. & Kitagawa, Y. Cloning and expression of Thiobacillus ferrooxidans mercury ion resistance genes in Escherichia coli. J. Bacteriol. 171, 3458–3464 (1989).
Wang, C. L., Maratukulam, P. D., Lum, A. M., Clark, D. S. & Keasling, J. D. Metabolic engineering of an aerobic sulfate reduction pathway and its application to precipitation of cadmium on the cell surface. Appl. Environ. Microbiol. 66, 4497–4502 (2000).
Krämer, U. & Chardonnens, A. The use of transgenic plants in the bioremediation of soils contaminated with trace elements. Appl. Microbiol. Biotechnol. 55, 661–672 (2001).
Sayler, G. S. & Ripp, S. Field applications of genetically engineered microorganisms for bioremediation processes. Curr. Opin. Biotechnol. 11, 286–289 (2000).
Vieira, É. D., da Graça Stupiello Andrietta, M. & Andrietta, S. R. Yeast biomass production: a new approach in glucose-limited feeding strategy. Braz. J. Microbiol. 44, 551–558 (2013).
Worldwide Beer Production, 2016 (Barth-Haas Group, 2018).
Value of the Yeast Product Market Worldwide from 2016 to 2022 (in Billions U.S. Dollars) (PR Newswire, 2017).
Swiegers, J. H. & Pretorius, I. S. Modulation of volatile sulfur compounds by wine yeast. Appl. Microbiol. Biotechnol. 74, 954–960 (2007).
Linderholm, A. L., Findleton, C. L., Kumar, G., Hong, Y. & Bisson, L. F. Identification of genes affecting hydrogen sulfide formation in Saccharomyces cerevisiae. Appl. Environ. Microbiol. 74, 1418–1427 (2008).
Rickard, D. & Luther, G. W. Metal sulfide complexes and clusters. Rev. Mineral. Geochem. 61, 421–504 (2006).
Bolong, N., Ismail, A. F., Salim, M. R. & Matsuura, T. A review of the effects of emerging contaminants in wastewater and options for their removal. Desalination 239, 229–246 (2009).
Johnson, D. B. & Hallberg, K. B. Acid mine drainage remediation options: a review. Sci. Total Environ. 338, 3–14 (2005).
Table of Regulated Drinking Water Contaminants (EPA, 2019).
Secondary Drinking Water Standards: Guidance for Nuisance Chemicals (EPA, 2019).
Sparks, B. D., Kotlyar, L. S., O’Carroll, J. B. & Chung, K. H. Athabasca oil sands: effect of organic coated solids on bitumen recovery and quality. J. Pet. Sci. Eng. 39, 417–430 (2003).
Kelly, E. N. et al. Oil sands development contributes elements toxic at low concentrations to the Athabasca River and its tributaries. Proc. Natl Acad. Sci. USA 107, 16178–16183 (2010).
Sakimoto, K. K., Wong, A. B. & Yang, P. Self-photosensitization of nonphotosynthetic bacteria for solar-to-chemical production. Science 351, 74–77 (2016).
Sweeney, R. Y. et al. Bacterial biosynthesis of cadmium sulfide nanocrystals. Chem. Biol. 11, 1553–1559 (2004).
DeSilva Tara, M., Gianluigi, Veglia, Fernando, Porcelli, Prantner Andrew, M. & Opella Stanley, J. Selectivity in heavy metal‐ binding to peptides and proteins. Biopolymers 64, 189–197 (2002).
Kim, J. et al. Effects of various pretreatments for enhanced anaerobic digestion with waste activated sludge. J. Biosci. Bioeng. 95, 271–275 (2003).
Harrison, R. G., Todd, P., Todd, P. W., Rudge, S. R. & Petrides, D. P. Bioseparations Science and Engineering (Oxford Univ. Press, 2015).
Hoek, P. V., Dijken, J. P. V. & Pronk, J. T. Effect of specific growth rate on fermentative capacity of baker’s yeast. Appl. Environ. Microbiol. 64, 4226–4233 (1998).
Yeasts, Yeast Extracts, Autolysates and Related Products: The Global Market (BCC Research, 2017).
Rugh, C. L. et al. Mercuric ion reduction and resistance in transgenic Arabidopsis thaliana plants expressing a modified bacterial merA gene. Proc. Natl Acad. Sci. USA 93, 3182–3187 (1996).
Arai, T. et al. Cu-doped ZnS hollow particle with high activity for hydrogen generation from alkaline sulfide solution under visible light. Chem. Mater. 20, 1997–2000 (2008).
Parveen, A., Agrawal, S. & Azam, A. Band gap tuning and fluorescence properties of lead sulfide Pb0.9A0.1S (A: Fe, Co, and Ni) nanoparticles by transition metal doping. Opt. Mater. 76, 21–27 (2018).
Bhattacharya, S. & Chakravorty, D. Electrical and magnetic properties of cold compacted iron-doped zinc sulfide nanoparticles synthesized by wet chemical method. Chem. Phys. Lett. 444, 319–323 (2007).
Selim, H., Gupta, A. K. & Al Shoaibi, A. Effect of reaction parameters on the quality of captured sulfur in Claus process. Appl. Energy 104, 772–776 (2013).
Minerals Information: Sulfur (USGS, 2018).
Little, B. J., Ray, R. I. & Pope, R. K. Relationship between corrosion and the biological sulfur cycle: a review. CORROSION 56, 433–443 (2000).
Seligman, A. M., Wasserkrug, H. L. & Hanker, J. S. A new staining method (OTO) for enhancing contrast of lipid-containing membranes and droplets in osmium tetroxide-fixed tissue with osmiophilic thiocarbohydrazide (TCH). J. Cell Biol. 30, 424–432 (1966).
Source: Resources - nature.com