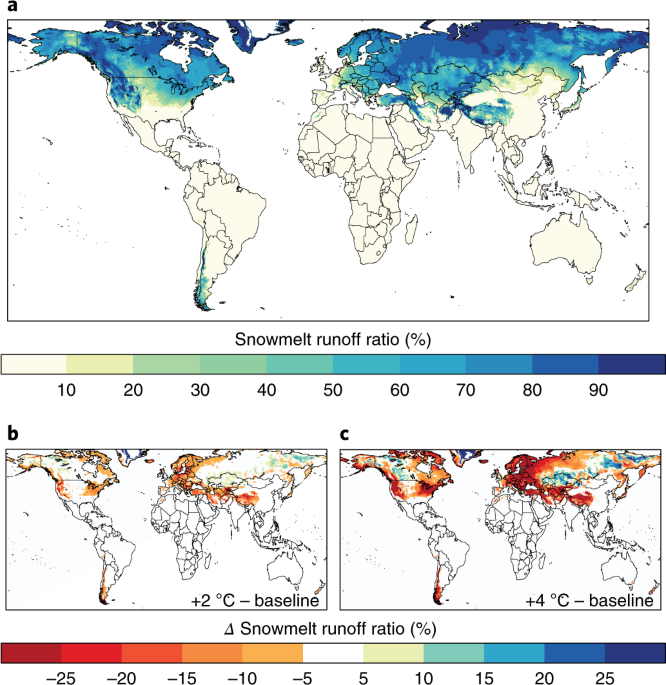
Brauman, K. A., Richter, B. D., Postel, S., Malsy, M. & Florke, M. Water depletion: an improved metric for incorporating seasonal and dry-year water scarcity into water risk assessments. Elem. Sci. Anth. 4, 000083 (2016).
Oki, T. & Kanae, S. Global hydrological cycles and world water resources. Science 313, 1068–1072 (2006).
Portmann, F. T., Siebert, S. & Doll, P. MIRCA2000—Global monthly irrigated and rainfed crop areas around the year 2000: a new high-resolution data set for agricultural and hydrological modeling. Global Biogeochem. Cycles 24, GB1011 (2010).
Bruinsma, J. (ed.) World Agriculture: Towards 2015/2030. An FAO Perspective (Earthscan, 2003).
Alexandratos, N. & Bruinsma, J. World Agriculture Towards 2030/2050: The 2012 Revision. ESA Working Paper No. 12-03 (FAO, 2012).
Siebert, S. et al. Groundwater use for irrigation—a global inventory. Hydrol. Earth Syst. Sci. 14, 1863–1880 (2010).
Jiménez Cisneros, B. E. et al. in Climate Change 2014: Impacts, Adaptation, and Vulnerability (eds, Field, C. B. et al) 229–269 (IPCC, Cambridge Univ. Press, 2014).
Elliott, J. et al. Constraints and potentials of future irrigation water availability on agricultural production under climate change. Proc. Natl Acad. Sci. USA 111, 3239–3244 (2014).
Yu, C. Q. et al. Assessing the impacts of extreme agricultural droughts in China under climate and socioeconomic changes. Earths Future 6, 689–703 (2018).
Vano, J. A. et al. Climate change impacts on water management and irrigated agriculture in the Yakima River Basin, Washington, USA. Clim. Change 102, 287–317 (2010).
Portmann, F., Siebert, S., Bauer, C. & Döll, P. Global Dataset of Monthly Growing Areas of 26 Irrigated Crops. Frankfurt Hydrology Paper 06. Institute of Physical Geography 400 (University of Frankfurt, 2008).
Waliser, D. et al. Simulating cold season snowpack: impacts of snow albedo and multi-layer snow physics. Clim. Change 109, 95–117 (2011).
Huning, L. S. & AghaKouchak, A. Mountain snowpack response to different levels of warming. Proc. Natl Acad. Sci. USA 115, 10932–10937 (2018).
Li, D. Y., Wrzesien, M. L., Durand, M., Adam, J. & Lettenmaier, D. P. How much runoff originates as snow in the western United States, and how will that change in the future? Geophys. Res. Lett. 44, 6163–6172 (2017).
Vicuna, S., McPhee, J. & Garreaud, R. D. Agriculture vulnerability to climate change in a snowmelt-driven basin in semiarid chile. J Water Resour. Plan. Manag. 138, 431–441 (2012).
Easterling, W. E. et al. in Climate Change 2007: Impacts, Adaptation and Vulnerability (Parry, M. L. et al.) 273–313 (IPCC, Cambridge Univ. Press, 2007).
Kapnick, S. B. & Delworth, T. L. Controls of global snow under a changed climate. J. Clim. 26, 5537–5562 (2013).
Barnett, T. P., Adam, J. C. & Lettenmaier, D. P. Potential impacts of a warming climate on water availability in snow-dominated regions. Nature 438, 303–309 (2005).
Adam, J. C., Hamlet, A. F. & Lettenmaier, D. P. Implications of global climate change for snowmelt hydrology in the twenty-first century. Hydrol. Process. 23, 962–972 (2009).
Immerzeel, W. W., van Beek, L. P. H. & Bierkens, M. F. P. Climate change will affect the asian water towers. Science 328, 1382–1385 (2010).
Mankin, J. S., Viviroli, D., Singh, D., Hoekstra, A. Y. & Diffenbaugh, N. S. The potential for snow to supply human water demand in the present and future. Environ. Res. Lett. 10, 114016 (2015).
Abatzoglou, J. T., Dobrowski, S. Z., Parks, S. A. & Hegewisch, K. C. Terraclimate, a high-resolution global dataset of monthly climate and climatic water balance from 1958-2015. Sci. Data 5, 170191 (2018).
Vorosmarty, C. J., Green, P., Salisbury, J. & Lammers, R. B. Global water resources: vulnerability from climate change and population growth. Science 289, 284–288 (2000).
Siebert, S. & Doll, P. Quantifying blue and green virtual water contents in global crop production as well as potential production losses without irrigation. J. Hydrol. 384, 198–217 (2010).
Food and Agriculture Data (Food and Agriculture Organization of the United Nations, 2018); http://www.fao.org/faostat/en/#data
Hoekstra, A. Y., Mekonnen, M. M., Chapagain, A. K., Mathews, R. E. & Richter, B. D. Global monthly water scarcity: blue water footprints versus blue water availability. PLoS ONE 7, e32688 (2012).
Goldewijk, K. K., Beusen, A., Doelman, J. & Stehfest, E. Anthropogenic land use estimates for the Holocene – HYDE 3.2. Earth Syst. Sci. Data 9, 927–953 (2017).
IPCC: Summary for Policymakers. In Climate Change 2014: Impacts, Adaptation, and Vulnerability (eds Field, C. B. et al.) 1–32 (Cambridge Univ. Press, 2014).
Sharma, J. & Ravindranath, N. Applying IPCC 2014 framework for hazard-specific vulnerability assessment under climate change. Environ. Res. Commun. 1, 051004 (2019).
Hagenlocher, M. et al. Drought vulnerability and risk assessments: state of the art, persistent gaps, and research agenda. Environ. Res. Lett. 14, 083002 (2019).
Ben Fraj, W., Elloumi, M. & Molle, F. The politics of interbasin transfers: socio-environmental impacts and actor strategies in Tunisia. Nat. Resour. Forum 43, 17–30 (2019).
Liu, L. et al. Quantifying the potential for reservoirs to secure future surface water yields in the world’s largest river basins. Environ. Res. Lett. 13, 044026 (2018).
Wada, Y. et al. Global monthly water stress: 2. Water demand and severity of water stress.Water Resour. Res. 47, W07518 (2011).
Nelson, K. S. & Burchfield, E. K. Effects of the structure of water rights on agricultural production during drought: a spatiotemporal analysis of California’s central valley. Water Resour. Res. 53, 8293–8309 (2017).
Lehner, B. C. et al. High-resolution mapping of the world’s reservoirs and dams for sustainable river-flow management. Front. Ecol. Environ. 9, 494–502 (2011).
Qin, Y. et al. Flexibility and intensity of global water use. Nat. Sustain. 2, 515–523 (2019).
Sneed M. & Brandt J. M. S. Land Subsidence Along the Delta–Mendota Canal in the Northern Part of the San Joaquin Valley, California, 2003–2010 Scientific Investigations Report 2013-5142 (US Geological Survey, 2013); http://pubs.usgs.gov/sir/2013/5142/
Godfray, H. C. J. et al. Food security: the challenge of feeding 9 billion people. Science 327, 812–818 (2010).
Myers, S. S. et al. Climate change and global food systems: potential impacts on food security and undernutrition. Annu. Rev. Publ. Health 38, 259–277 (2017).
Pritchard, H. D. Asia’s shrinking glaciers protect large populations from drought stress. Nature 569, 649–654 (2019).
Mankin, J. S. & Diffenbaugh, N. S. Influence of temperature and precipitation variability on near-term snow trends. Clim. Dynam. 45, 1099–1116 (2015).
Rothausen, S. G. S. A. & Conway, D. Greenhouse-gas emissions from energy use in the water sector. Nat. Clim. Change 1, 210–219 (2011).
Schewe, J. et al. Multimodel assessment of water scarcity under climate change. Proc. Natl Acad. Sci. USA 111, 3245–3250 (2014).
Bormann, K. J., Brown, R. D., Derksen, C. & Painter, T. H. Estimating snow-cover trends from space. Nat. Clim. Change 8, 923–927 (2018).
Knowles, N., Dettinger, M. D. & Cayan, D. R. Trends in snowfall versus rainfall in the Western United States. J. Clim. 19, 4545–4559 (2006).
Mote, P. W. Trends in snow water equivalent in the Pacific Northwest and their climatic causes. Geophys. Res. Lett. 30, 12 (2003).
Westerling, A. L., Hidalgo, H. G., Cayan, D. R. & Swetnam, T. W. Warming and earlier spring increase western US forest wildfire activity. Science 313, 940–943 (2006).
Davis, S. J. et al. Net-zero emissions energy systems. Science 360, eaas9793 (2018).
Guan, D. B. et al. Structural decline in China’s CO2 emissions through transitions in industry and energy systems. Nat. Geosci. 11, 551–555 (2018).
Davies, D. M. et al. Combined economic and technological evaluation of battery energy storage for grid applications. Nat. Energy 4, 42–50 (2019).
Clow, D. W. Changes in the timing of snowmelt and streamflow in colorado: A response to recent warming. J Clim. 23, 2293–2306 (2010).
Rasmussen, R. et al. Climate change impacts on the water balance of the colorado headwaters: high-resolution regional climate model simulations. J. Hydrometeorol. 15, 1091–1116 (2014).
The NCAR Command Language v.6. 6.2 (NCAR, 2019); https://doi.org/10.5065/D6WD3XH5
Snow Data Assimilation System (SNODAS) Data Products at NSIDC, Version 1 (NSIDC, accessed December 2018); https://doi.org/10.7265/N5TB14TC
Mitchell, T. D. Pattern scaling—an examination of the accuracy of the technique for describing future climates. Clim. Change 60, 217–242 (2003).
Huntingford, C. & Cox, P. M. An analogue model to derive additional climate change scenarios from existing GCM simulations. Clim. Dynam. 16, 575–586 (2000).
Kirtman, B. et al. in Climate Change 2013: The Physical Science Basis (eds Stocker, T. F. et al.) Ch. 11 (IPCC, Cambridge Univ. Press, 2013).
Hawkins, E. et al. Estimating changes in global temperature since the preindustrial period. Bull. Am. Meteorol. Soc. 98, 1841–1856 (2017).
James, R., Washington, R., Schleussner, C. F., Rogelj, J. & Conway, D. Characterizing half-a-degree difference: a review of methods for identifying regional climate responses to global warming targets. WIRES Clim. Change 8, e457 (2017).
Kruijt, B., Witte, J. P. M., Jacobs, C. M. J. & Kroon, T. Effects of rising atmospheric CO2 on evapotranspiration and soil moisture: a practical approach for the Netherlands. J. Hydrol. 349, 257–267 (2008).
Source: Resources - nature.com