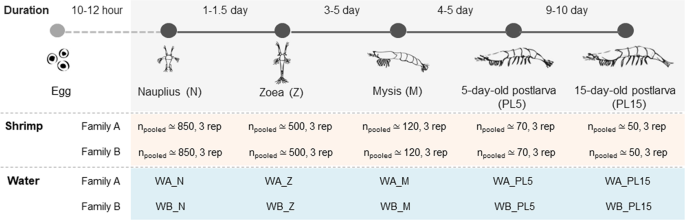
Buchon, N., Broderick, N. A. & Lemaitre, B. Gut homeostasis in a microbial world: insights from Drosophila melanogaster. Nat. Rev. Microbiol. 11, 615, https://doi.org/10.1038/nrmicro3074 (2013).
Hooper, L. V., Midtvedt, T. & Gordon, J. I. How host-microbial interactions shape the nutrient environment of the mammalian intestine. Annu. Rev. Nutr. 22, 283–307, https://doi.org/10.1146/annurev.nutr.22.011602.092259 (2002).
Rawls, J. F., Samuel, B. S. & Gordon, J. I. Gnotobiotic zebrafish reveal evolutionarily conserved responses to the gut microbiota. Proc. Natl Acad. Sci. USA 101, 4596–4601, https://doi.org/10.1073/pnas.0400706101 (2004).
Arrieta, M. C., Stiemsma, L. T., Amenyogbe, N., Brown, E. M. & Finlay, B. The intestinal microbiome in early life: health and disease. Front. Immunol. 5, 427–427, https://doi.org/10.3389/fimmu.2014.00427 (2014).
Belkaid, Y. & Hand, T. W. Role of the microbiota in immunity and inflammation. Cell 157, 121–141, https://doi.org/10.1016/j.cell.2014.03.011 (2014).
Pickard, J. M., Zeng, M. Y., Caruso, R. & Núñez, G. Gut microbiota: Role in pathogen colonization, immune responses, and inflammatory disease. Immunol. Rev. 279, 70–89, https://doi.org/10.1111/imr.12567 (2017).
Chaiyapechara, S. et al. Bacterial community associated with the intestinal tract of P. monodon in commercial farms. Microb. Ecol. 63, 938–953, https://doi.org/10.1007/s00248-011-9936-2 (2011).
Rungrassamee, W. et al. Bacterial population in intestines of the black tiger shrimp (Penaeus monodon) under different growth stages. Plos One 8, https://doi.org/10.1371/journal.pone.0060802 (2013).
Rungrassamee, W. et al. Characterization of intestinal bacteria in wild and domesticated adult black tiger shrimp (Penaeus monodon). Plos One 9, https://doi.org/10.1371/journal.pone.0091853 (2014).
Rungrassamee, W., Klanchui, A., Maibunkaew, S. & Karoonuthaisiri, N. Bacterial dynamics in intestines of the black tiger shrimp and the Pacific white shrimp during Vibrio harveyi exposure. J. Invertebr. Pathol. 133, 12–19, https://doi.org/10.1016/j.jip.2015.11.004 (2016).
Gomez-Gil, B., Roque, A. & Turnbull, J. F. The use and selection of probiotic bacteria for use in the culture of larval aquatic organisms. Aquaculture 191, 259–270, https://doi.org/10.1016/S0044-8486(00)00431-2 (2000).
Newaj-Fyzul, A., Al-Harbi, A. H. & Austin, B. Review: Developments in the use of probiotics for disease control in aquaculture. Aquaculture 431, 1–11, https://doi.org/10.1016/j.aquaculture.2013.08.026 (2014).
Ringø, E. et al. Effect of dietary components on the gut microbiota of aquatic animals. A never-ending story? Aquac. Nutr. 22, 219–282, https://doi.org/10.1111/anu.12346 (2015).
Motoh, H. Biology and ecology of Penaeus monodon. Iloilo City, Philippines: Aquaculture Department, Southeast Asian Fisheries Development Center, 27–36 (1985).
Jiravanichpaisal, P. et al. Expression of immune-related genes in larval stages of the giant tiger shrimp, Penaeus monodon. Fish. Shellfish. Immunol. 23, 815–824, https://doi.org/10.1016/j.fsi.2007.03.003 (2007).
Bates, J. M. et al. Distinct signals from the microbiota promote different aspects of zebrafish gut differentiation. Dev. Biol. 297, 374–386, https://doi.org/10.1016/j.ydbio.2006.05.006 (2006).
Tamburini, S., Shen, N., Wu, H. C. & Clemente, J. C. The microbiome in early life: implications for health outcomes. Nat. Med. 22, 713–722, https://doi.org/10.1038/nm.4142 (2016).
Xia, Y. & Sun, J. Hypothesis testing and statistical analysis of microbiome. Genes. Dis. 4, 138–148, https://doi.org/10.1016/j.gendis.2017.06.001 (2017).
Stephens, W. Z. et al. The composition of the zebrafish intestinal microbial community varies across development. ISME J. 10, 644–654, https://doi.org/10.1038/ismej.2015.140 (2016).
Egerton, S., Culloty, S., Whooley, J., Stanton, C. & Ross, R. P. The gut microbiota of marine fish. Front. Microbiol. 9, 873–873, https://doi.org/10.3389/fmicb.2018.00873 (2018).
Fan, L. et al. Microbiota comparison of Pacific white shrimp intestine and sediment at freshwater and marine cultured environment. Sci. Total. Env. 657, 1194–1204, https://doi.org/10.1016/j.scitotenv.2018.12.069 (2019).
Tanaka, M. & Nakayama, J. Development of the gut microbiota in infancy and its impact on health in later life. Allergol. Int. 66, 515–522, https://doi.org/10.1016/j.alit.2017.07.010 (2017).
Lloyd-Price, J., Abu-Ali, G. & Huttenhower, C. The healthy human microbiome. Genome Med. 8, 51–51, https://doi.org/10.1186/s13073-016-0307-y (2016).
Mongkol, P. et al. Bacterial community composition and distribution in different segments of the gastrointestinal tract of wild‐caught adult Penaeus monodon. Aquac. Res. 49, 378–392, https://doi.org/10.1111/are.13468 (2017).
Pangastuti, A., Suwanto, A., Lestari, Y. & Suhartono, M. Bacterial communities associated with white shrimp (Litopenaeus vannamei) larvae at early developmental stages. Biodiversitas 11, 65–68, https://doi.org/10.13057/biodiv/d110203 (2010).
Huang, Z., Li, X., Wang, L. & Shao, Z. Changes in the intestinal bacterial community during the growth of white shrimp, Litopenaeus vannamei. Aquac. Res. 47, 1737–1746, https://doi.org/10.1111/are.12628 (2016).
Zheng, Y. et al. Comparison of cultivable bacterial communities associated with Pacific white shrimp (Litopenaeus vannamei) larvae at different health statuses and growth stages. Aquaculture 451, 163–169, https://doi.org/10.1016/j.aquaculture.2015.09.020 (2016).
Zheng, Y. et al. Bacterial community associated with healthy and diseased Pacific white shrimp (Litopenaeus vannamei) larvae and rearing water across different growth stages. Front. Microbiol. 8, 1362–1362, https://doi.org/10.3389/fmicb.2017.01362 (2017).
Roeselers, G. et al. Evidence for a core gut microbiota in the zebrafish. ISME J. 5, 1595–1608, https://doi.org/10.1038/ismej.2011.38 (2011).
Thompson, F. L., Iida, T. & Swings, J. Biodiversity of vibrios. Microbiol. Mol. Biol. Rev. 68, 403–431, https://doi.org/10.1128/MMBR.68.3.403-431.2004 (2004).
Ortigosa, M., Garay, E. & Pujalte, M. J. Numerical taxonomy of Vibrionaceae isolated from oysters and seawater along an annual cycle. Syst. Appl. Microbiol. 17, 216–225, https://doi.org/10.1016/S0723-2020(11)80011-1 (1994).
Vandenberghe, J. et al. Vibrios Associated with Litopenaeus vannamei Larvae, Postlarvae, Broodstock, and Hatchery Probionts. Appl. Env. Microbiol. 65, 2592–2597 (1999).
Crenn, K., Duffieux, D. & Jeanthon, C. Bacterial epibiotic communities of ubiquitous and abundant marine diatoms are distinct in short- and long-term associations. Front. Microbiol. 9, 2879–2879, https://doi.org/10.3389/fmicb.2018.02879 (2018).
Fuerst, J. A. et al. Isolation and molecular identification of planctomycete bacteria from postlarvae of the giant tiger prawn, Penaeus monodon. Appl. Env. Microbiol. 63, 254–262 (1997).
Li, M. & Gu, J. D. The diversity and distribution of anammox bacteria in the marine aquaculture zones. Appl. Microbiol. Biotechnol. 100, 8943–8953, https://doi.org/10.1007/s00253-016-7690-6 (2016).
Crab, R., Avnimelech, Y., Defoirdt, T., Bossier, P. & Verstraete, W. Nitrogen removal techniques in aquaculture for a sustainable production. Aquaculture 270, 1–14, https://doi.org/10.1016/j.aquaculture.2007.05.006 (2007).
Cornejo-Granados, F., Gallardo-Becerra, L., Leonardo-Reza, M., Ochoa-Romo, J. P. & Ochoa-Leyva, A. A meta-analysis reveals the environmental and host factors shaping the structure and function of the shrimp microbiota. PeerJ 6, 5382–5382, https://doi.org/10.7717/peerj.5382 (2018).
Ronquillo, J. D., Saisho, T. & McKinley, R. S. Early developmental stages of the green tiger prawn, Penaeus semisulcatus de Haan (Crustacea, Decapoda, Penaeidae). Hydrobiologia 560, 175–196, https://doi.org/10.1007/s10750-005-1448-y (2006).
Hassan, H.-U. The larval development of Penaeus semisulcatus de Haan, 1850 (Decapoda, Penaeidae) reared in the laboratory. J. Plankton Res. 4, 1–17, https://doi.org/10.1093/plankt/4.1.1 (1982).
Hammer, T. J., McMillan, W. O. & Fierer, N. Metamorphosis of a butterfly-associated bacterial community. Plos One 9, https://doi.org/10.1371/journal.pone.0086995 (2014).
Chen, B. et al. Gut bacterial and fungal communities of the domesticated silkworm (Bombyx mori) and wild mulberry-feeding relatives. ISME J. 12, 2252–2262, https://doi.org/10.1038/s41396-018-0174-1 (2018).
Landsman, A., St-Pierre, B., Rosales-Leija, M., Brown, M. & Gibbons, W. Investigation of the potential effects of host genetics and probiotic treatment on the gut bacterial community composition of aquaculture-raised Pacific whiteleg shrimp, Litopenaeus vannamei. Microorg. 7, 217, https://doi.org/10.3390/microorganisms7080217 (2019).
Fan, J. et al. Dynamics of the gut microbiota in developmental stages of Litopenaeus vannamei reveal its association with body weight. Sci. Rep. 9, 734–734, https://doi.org/10.1038/s41598-018-37042-3 (2019).
Hasan, N. & Yang, H. Factors affecting the composition of the gut microbiota, and its modulation. PeerJ 7, 7502–7502, https://doi.org/10.7717/peerj.7502 (2019).
Scepanovic, P. et al. A comprehensive assessment of demographic, environmental, and host genetic associations with gut microbiome diversity in healthy individuals. Microbiome 7, 130, https://doi.org/10.1186/s40168-019-0747-x (2019).
Rothschild, D. et al. Environment dominates over host genetics in shaping human gut microbiota. Nat. 555, 210, https://doi.org/10.1038/nature25973 (2018).
Cornejo-Granados, F. et al. Microbiome of Pacific Whiteleg shrimp reveals differential bacterial community composition between Wild, Aquacultured and AHPND/EMS outbreak conditions. Sci. Rep. 7, 11783, https://doi.org/10.1038/s41598-017-11805-w (2017).
Collado, M. C., Cernada, M., Baüerl, C., Vento, M. & Pérez-Martínez, G. Microbial ecology and host-microbiota interactions during early life stages. Gut Microbes 3, 352–365, https://doi.org/10.4161/gmic.21215 (2012).
Kaczmarczyk, A. et al. First insight into microbiome profile of fungivorous thrips Hoplothrips carpathicus (Insecta: Thysanoptera) at different developmental stages: molecular evidence of Wolbachia endosymbiosis. Sci. Rep. 8, 14376–14376, https://doi.org/10.1038/s41598-018-32747-x (2018).
Pérez, T. et al. Host–microbiota interactions within the fish intestinal ecosystem. Mucosal Immunol. 3, 355, https://doi.org/10.1038/mi.2010.12 (2010).
Balcázar, J. L., Rojas-Luna, T. & Cunningham, D. P. Effect of the addition of four potential probiotic strains on the survival of pacific white shrimp (Litopenaeus vannamei) following immersion challenge with Vibrio parahaemolyticus. J. Invertebr. Pathol. 96, 147–150, https://doi.org/10.1016/j.jip.2007.04.008 (2007).
Verschuere, L., Rombaut, G., Sorgeloos, P. & Verstraete, W. Probiotic bacteria as biological control agents in aquaculture. Microbiol. Mol. Biol. Rev. 64, 655–671, https://doi.org/10.1128/mmbr.64.4.655-671.2000 (2000).
Chen, W.-Y., Ng, T. H., Wu, J.-H., Chen, J.-W. & Wang, H.-C. Microbiome dynamics in a shrimp grow-out pond with possible outbreak of acute hepatopancreatic necrosis disease. Sci. Rep. 7, 9395–9395, https://doi.org/10.1038/s41598-017-09923-6 (2017).
Ridgway, I. D. et al. Extracellular proteases and possible disease related virulence mechanisms of two marine bacteria implicated in an opportunistic bacterial infection of Nephrops norvegicus. J. Invertebr. Pathol. 99, 14–19, https://doi.org/10.1016/j.jip.2008.05.007 (2008).
Sorieul, L. et al. Survival improvement conferred by the Pseudoalteromonas sp. NC201 probiotic in Litopenaeus stylirostris exposed to Vibrio nigripulchritudo infection and salinity stress. Aquaculture 495, 888–898, https://doi.org/10.1016/j.aquaculture.2018.06.058 (2018).
Klindworth, A. et al. Evaluation of general 16S ribosomal RNA gene PCR primers for classical and next-generation sequencing-based diversity studies. Nucleic Acids Res 41, https://doi.org/10.1093/nar/gks808 (2013).
Bacchetti De Gregoris, T., Aldred, N., Clare, A. S. & Burgess, J. G. Improvement of phylum- and class-specific primers for real-time PCR quantification of bacterial taxa. J. Microbiol. Meth 86, 351–356, https://doi.org/10.1016/j.mimet.2011.06.010 (2011).
Fierer, N., Jackson, J. A., Vilgalys, R. & Jackson, R. B. Assessment of soil microbial community structure by use of taxon-specific quantitative PCR assays. Appl. Env. Microbiol. 71, 4117–4120, https://doi.org/10.1128/AEM.71.7.4117-4120.2005 (2005).
FastQC: a quality control tool for high throughput sequence data (2010).
Ewels, P., Magnusson, M., Käller, M. & Lundin, S. MultiQC: summarize analysis results for multiple tools and samples in a single report. Bioinforma. 32, 3047–3048, https://doi.org/10.1093/bioinformatics/btw354 (2016).
Schloss, P. D. et al. Introducing mothur: open-source, platform-independent, community-supported software for describing and comparing microbial communities. Appl. Env. Microbiol. 75, 7537–7541, https://doi.org/10.1128/AEM.01541-09 (2009).
Kozich, J. J., Westcott, S. L., Baxter, N. T., Highlander, S. K. & Schloss, P. D. Development of a dual-index sequencing strategy and curation pipeline for analyzing amplicon sequence data on the MiSeq Illumina sequencing platform. Appl. Env. Microbiol. 79, 5112–5120, https://doi.org/10.1128/AEM.01043-13 (2013).
Parte, A. C. LPSN – List of Prokaryotic names with Standing in Nomenclature (bacterio.net), 20 years on. Int. J. Syst. Evol. Microbiol. 68, 1825–1829, https://doi.org/10.1099/ijsem.0.002786 (2018).
Cole, J. R. et al. Ribosomal Database Project: data and tools for high throughput rRNA analysis. Nucleic Acids Res. 42, 633–642, https://doi.org/10.1093/nar/gkt1244 (2014).
Dixon, P. VEGAN, a package of R functions for community ecology. Vol. 14 (2003).
McMurdie, P. J. & Holmes, S. phyloseq: an R package for reproducible interactive analysis and graphics of microbiome census data. Plos One 8, https://doi.org/10.1371/journal.pone.0061217 (2013).
R: A language and environment for statistical computing (R Foundation for Statistical Computing, Vienna, Austria, 2018).
RStudio: Integrated Development for R (RStudio, Inc., Boston, MA, 2015).
Close, R., Evers, S., Alroy, J. & Butler, R. How should we estimate diversity in the fossil record? Testing richness estimators using sampling-standardised discovery curves. Methods Ecol Evol 9, https://doi.org/10.1111/2041-210X.12987 (2018).
Good, I. J. The population frequencies of species and the estimation of population parameters. Biometrika 40, 237–264, https://doi.org/10.2307/2333344 (1953).
Andersen, K. S., Kirkegaard, R. H., Karst, S. M. & Albertsen, M. ampvis2: an R package to analyse and visualise 16S rRNA amplicon data. bioRxiv, 299537, https://doi.org/10.1101/299537 (2018).
Altschul, S. F., Gish, W., Miller, W., Myers, E. W. & Lipman, D. J. Basic local alignment search tool. J. Mol. Biol. 215, 403–410, https://doi.org/10.1016/S0022-2836(05)80360-2 (1990).
Source: Ecology - nature.com