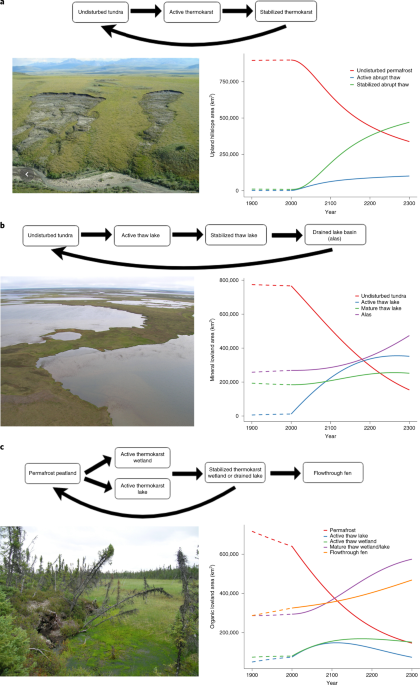
Schuur, E. A. G. et al. Climate change and the permafrost carbon feedback. Nature 520, 171–179 (2015).
McGuire, A. D. et al. Dependence of the evolution of carbon dynamics in the northern permafrost region on the trajectory of climate change. Proc. Natl Acad. Sci. USA 115, 3882–3887 (2018).
Hugelius, G. et al. Estimated stocks of circumpolar permafrost carbon with quantified uncertainty ranges and identified data gaps. Biogeosciences 11, 6573–6593 (2014).
Schuur, E. A. G., McGuire, A. D., Romanovsky, V., Schädel, C. & Mack, M. in Second State of the Carbon Cycle Report (SOCCR2): A Sustained Assessment Report (eds Cavallaro, N. et al.) 428–468 (US Global Change Research Program, 2018).
Schuur, E. A. G. et al. Vulnerability of permafrost carbon to climate change: implications for the global carbon cycle. Bioscience 58, 701–714 (2008).
Khvorostyanov, D. V. et al. Vulnerability of permafrost carbon to global warming. Part II: sensitivity of permafrost carbon stock to global warming. Tellus B Chem. Phys. Meteorol. 60, 265–275 (2007).
Tarnocai, C. The effect of climate warming on the carbon balance of cryosols in Canada. Permafrost Periglac. Process. 10, 251–263 (1999).
Zimov, S. A. et al. Permafrost carbon: stock and decomposability of a globally significant carbon pool. Geophys. Res. Lett. 33, L20502 (2006).
Schaefer, K., Lantuit, H., Romanovsky, V. E., Schuur, E. A. G. & Witt, R. The impact of the permafrost carbon feedback on global climate. Environ. Res. Lett. 9, 085003 (2014).
Abbott, B. W. et al. Biomass offsets little or none of permafrost carbon release from soils, streams, and wildfire: an expert assessment. Environ. Res. Lett. 11, 034014 (2016).
Lawrence, D. M., Koven, C. D., Swenson, S. C., Riley, W. J. & Slater, A. G. Permafrost thaw and resulting soil moisture changes regulate projected high-latitude CO2 and CH4 emissions. Environ. Res. Lett. 10, 094011 (2015).
Koven, C. D. et al. A simplified, data-constrained approach to estimate the permafrost carbon–climate feedback. Phil. Trans. R. Soc. A Math. Phys. Eng. Sci. 373, 20140423 (2015).
MacDougall, A. H., Avis, C. A. & Weaver, A. J. Significant existing commitment to warming from the permafrost carbon feedback. Nat. Geosci. 5, 719–721 (2012).
Olefeldt, D. et al. Circumpolar distribution and carbon storage of thermokarst landscapes. Nat. Commun. 7, 13043 (2016).
Grosse, G. et al. Vulnerability of high-latitude soil organic carbon in North America to disturbance. J. Geophys. Res. 116, G00K06 (2011).
Kokelj, S. V. & Jorgenson, M. T. Advances in thermokarst research. Permafrost Periglac. Process. 24, 108–119 (2013).
Walter Anthony, K. M. et al. A shift of thermokarst lakes from carbon sources to sinks during the holocene epoch. Nature 511, 452–456 (2014).
Walter Anthony, K. M. et al. 21st-century modeled permafrost carbon emissions accelerated by abrupt thaw beneath lakes. Nat. Commun. 9, 3262 (2018).
Schneider von Deimling, T. et al. Observation-based modelling of permafrost carbon fluxes with accounting for deep carbon deposits and thermokarst activity. Biogeosciences 12, 3469–3488 (2015).
Turetsky, M. R. et al. Permafrost collapse is accelerating carbon release. Nature 569, 32–34 (2019).
Houghton, R. A. et al. Changes in the carbon content of terrestrial biota and soils between 1860–1980. Ecol. Monogr. 53, 235–262 (1983).
Treat, C. C. et al. Effects of permafrost aggradation on peat properties as determined from a pan-Arctic synthesis of plant macrofossils. J. Geophys. Res. 121, 78–94 (2016).
Lewkowicz, A. G. & Way, R. G. Extremes of summer climate trigger thousands of thermokarst landslides in a High Arctic environment. Nat. Commun. 10, 1329 (2019).
Jones, M. K. W., Pollard, W. H. & Jones, B. M. Rapid initialization of retrogressive thaw slumps in the Canadian High Arctic and their response to climate and terrain factors. Environ. Res Lett. 14, 055006 (2019).
Farquharson, L. M. et al. Climate change drives widespread and rapid thermokarst development in very cold permafrost in the Canadian High Arctic. Geophys. Res. Lett. 46, 6681–6689 (2019).
Schädel, C. et al. Potential carbon emissions dominated by carbon dioxide from thawed permafrost soils. Nat. Clim. Change 6, 950–953 (2016).
Serikova, S. et al. High riverine CO2 emissions at the permafrost boundary of western Siberia. Nat. Geosci. 11, 825–829 (2018).
Vonk, J. E. et al. High biolability of ancient permafrost carbon upon thaw. Geophys. Res. Lett. 40, 2689–2693 (2013).
Sannel, A. B. K. & Kuhry, P. Warming-induced destabilization of peat plateau/thermokarst lake complexes. J. Geophys. Res. 116, G03035 (2011).
Schuur, E. A. G. et al. Expert assessment of vulnerability of permafrost carbon to climate change. Clim. Change 119, 359–374 (2013).
Kleinen, T. & Brovkin, V. Pathway-dependent fate of permafrost region carbon. Environ. Res. Lett. 13, 094001 (2018).
Rogelj, J. et al. in Special Report on Global Warming of 1.5 °C (eds Masson-Delmotte, V. et al.) 93–174 (WMO, 2018).
Balser, A. W., Jones, J. B. & Gens, R. Timing of retrogressive thaw slump initiation in the Noatak Basin, northwest Alaska, USA. J. Geophys. Res. 119, 1106–1120 (2014).
Loranty, M. M. et al. Reviews and syntheses: changing ecosystem influences on soil thermal regimes in northern high-latitude permafrost regions. Biogeosciences 15, 5287–5313 (2018).
Baltzer, J. L., Veness, T., Chasmer, L. E., Sniderhan, A. E. & Quinton, W. L. Forests on thawing permafrost: fragmentation, edge effects, and net forest loss. Glob. Change Biol. 20, 824–834 (2014).
Kokelj, S. V., Lantz, T. C., Tunnicliffe, J., Segal, R. & Lacelle, D. Climate-driven thaw of permafrost preserved glacial landscapes, northwestern Canada. Geology 45, 371–374 (2017).
Walter Anthony, K. M. et al. Methane emissions proportional to permafrost carbon thawed in arctic lakes since the 1950s. Nat. Geosci. 9, 679–682 (2016).
Petrenko, V. et al. Minimal geological methane emissions during the younger dryas–preboreal abrupt warming event. Nature 548, 443–446 (2017).
Beck, J. et al. Bipolar carbon and hydrogen isotope constraints on the Holocene methane budget. Biogeosciences 15, 7155–7175 (2018).
Kruse, S. et al. Dispersal distances and migration rates at the arctic treeline in Siberia—a genetic and simulation-based study. Biogeosciences 16, 1211–1224 (2019).
Nitze, I., Grosse, G., Jones, B. M., Romanovsky, V. E. & Boike, J. Remote sensing quantifies widespread abundance of permafrost region disturbances across the Arctic and Subarctic. Nat. Commun. 9, 5423 (2018).
Abbott, B. W., Jones, J. B., Godsey, S. E., Larouche, J. R. & Bowden, W. B. Patterns and persistence of hydrologic carbon and nutrient export from collapsing upland permafrost. Biogeosciences 12, 3725–3740 (2015).
Tanski, G. et al. Transformation of terrestrial organic matter along thermokarst-affected permafrost coasts in the Arctic. Sci. Total Environ. 581–582, 434–447 (2017).
Estop-Aragones, C. et al. Respiration of aged soil carbon during fall in permafrost peatlands enhanced by active layer deepening following wildfire but limited following thermokarst. Environ. Res. Lett. 13, 085002 (2018).
O’Donnell, J. A. et al. The effects of permafrost thaw on soil hydrologic, thermal, and carbon dynamics in an Alaskan peatland. Ecosystems 15, 213–229 (2012).
Jones, M. C. et al. Rapid carbon loss and slow recovery following permafrost thaw in boreal peatlands. Glob. Change Biol. 23, 1109–1127 (2017).
Soetaert, K. & Petzoldt, T. Inverse modeling, sensitivity, and Monte Carlo analysis in R using package FME. J. Stat. Softw. https://doi.org/10.18637/jss.v033.i03 (2010)..
Myhre, G. et al. in Climate Change 2013: The Physical Science Basis (eds Stocker, T. F. et al.) 659–740 (Cambridge Univ. Press, 2013).
Frolking, S. & Roulet, N. T. Holocene radiative forcing impact of northern peatland carbon accumulation and methane emissions. Glob. Change Biol. 13, 1079–1088 (2007).
Frolking, S., Roulet, N. & Fuglestvedt, J. How northern peatlands influence the Earth’s radiative budget: sustained methane emission versus sustained carbon sequestration. J. Geophys. Res. 111, G01008 (2006).
Source: Ecology - nature.com