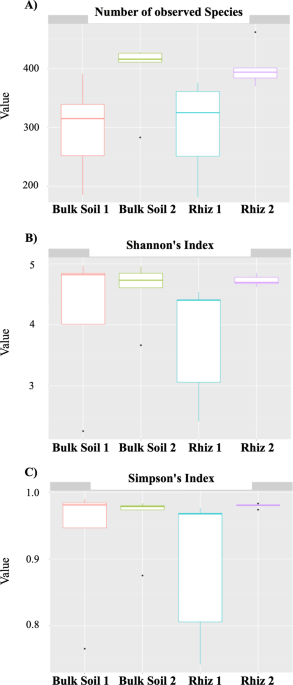
International Organisation of Vine and Wine Intergovernmental Organisation (OIV) 2019 Statistical Report on World Vitiviniculture, http://www.oiv.int/public/medias/6782/oiv-2019-statistical-report-on-world-vitiviniculture.pdf (2019).
International Organisation of Vine and Wine Intergovernmental Organisation (OIV) Distribution of the world’s grapevine varieties, http://www.oiv.int/public/medias/5888/en-distribution-of-the-worlds-grapevine-varieties.pdf (2017).
Vitulo, N. et al. A deep survey of alternative splicing in grape reveals changes in the splicing machinery related to tissue, stress condition and genotype BMC Plant Biol. 14, Article Number: 99 (2014).
Prezelj, N. et al. Metabolic consequences of infection of grapevine (Vitis vinifera L.) cv. “Modra frankinja” with Flavescence Doree Phytoplasma. Front. Plant Sci. 7, Article Number: 711 (2016).
Savoi, S. et al. Transcriptome and metabolite profiling reveals that prolonged drought modulates the phenylpropanoid and terpenoid pathway in white grapes (Vitis vinifera L.). BMC Plant Biol. 16, Article Number: 67 (2016).
Yildirim, K., Yagci, A., Sucu, S. & Tunc, S. Responses of grapevine rootstocks to drought through altered root system architecture and root transcriptomic regulations. Plant Physiol. Biochem. 127, 256–268 (2018).
Rosenberrg, I. Z. & Rosenberg, E. Role of microorganisms in the evolution of animals and plants: the hologenome theory of evolution. FEMS Microbiol. Rev. 32, 723–735 (2008).
Vandenkoornhuyse, P., Quaiser, A., Duhamel, M., Le Van, A. & Dufresne, A. The importance of the microbiome of the plant holobiont. New Phytol. 206, 1196–1206 (2015).
Bonfante, A. et al. A dynamic viticultural zoning to explore the resilience of terroir concept under climate change. Sci. Tot. Environ. 624, 294–308 (2018).
Cheng, G., He, Y.-N., Yue, T.-X., Wang, J. & Zhang, Z.-W. Effects of climatic conditions and soil properties on Cabernet Sauvignon berry growth and anthocyanin profiles. Molecules 19, 13683–13703 (2014).
Zerihun, A., McClymont, L., Lanyon, D., Goodwin, I. & Gibberd, M. Deconvoluting effects of vine and soil properties on grape berry composition. J. Sci. Food Agric. 95, 193–203 (2015).
Berlanas, C. et al. The fungal and bacterial rhizosphere microbiome associated with grapevine rootstock genotypes in mature and young vineyards. Front. Microbiol. 10, 1142, https://doi.org/10.3389/fmicb.2019.01142 (2019).
Marasco, R., Rolli, E., Fusi, M., Michoud, G. & Daffonchio, D. Grapevine rootstocks shape underground bacterial microbiome and networking but not potential functionality. Microbiome 6, 3, https://doi.org/10.1186/s40168-017-0391-2 (2018).
Lazcano, C., Gomez-Brandon, M., Revilla, P. & Dominguez, J. Short-term effects of organic and inorganic fertilizers on soil microbial community structure and function. Biol. Fertil. Soils 49, 723–733 (2013).
Vitulo, N. et al. Bark and grape microbiome of Vitis vinifera: influence of geographic patterns and agronomic management on bacterial diversity. Front. Microbiol. 9, 3203, https://doi.org/10.3389/fmicb.2018.03203 (2019).
Grangeteau, C. et al. Wine microbiology is driven by vineyard and winery anthropogenic factors. Microbiol. Biotechnol. 10, 354–370 (2017).
Bokulich, N. A., Thorngate, J. H., Richardson, P. M. & Mills, D. A. Microbial biogeography of wine grapes is conditioned by cultivar, vintage, and climate. PNAS USA 111, E139–E148 (2014).
Van Leeuwen, C. & Seguin, G. The concept of terroir in viticulture. J. Wine Res. 17, 1–10 (2006).
Gladstones, J. Introduction and definition of terroir. In: Wine, Terroir and Climate Change, ed. M. Deves (Kent Town, SA: Wakefield Press), 1-4 (2011).
Sigler, W. V. & Turco, R. F. The impact of chlorothalonil application on soil bacterial and fungal populations as assessed by denaturing gradient gel electrophoresis. Appl. Soil Ecol. 21, 107–118 (2002).
Rivera-Becerril, F. et al. Impact of a pesticide cocktail (fenhexamid, folpel, deltamethrin) on the abundance of Glomeromycota in two agricultural soils. Sci. Tot. Environ. 577, 84–93 (2017).
Muñoz-Leoz, B., Garbisu, C., Antigüedad, I. & Ruiz-Romera, E. Fertilization can modify the non-target effects of pesticides on soil microbial communities. Soil Biol. Biochem. 48, 125–134 (2012).
Canfora, L. et al. Vineyard microbiome variations during different fertilisation practices revealed by 16s rRNA gene sequencing. Appl. Soil Ecol. 125, 71–80 (2018).
Chi-Chu, L. Effect of pesticides on soil microbial community. J. Environ. Sci. Health, Part B 45, 348–359 (2010).
Novello, G. et al. The rhizosphere bacterial microbiota of Vitis vinifera cv. Pinot Noir in an Integrated Pest Management vineyard. Front. Microbiol. 8, 1528 (2017).
Bona, E. et al. A metaproteomic characterization of the Vitis vinifera rhizosphere. FEMS Microbiol. Ecol. 95, 1–16 (2019).
Rodriguez-R., L. M. & Konstantinidis, K. T. Estimating coverage in metagenomic data sets and why it matters. ISME J. 8, 2349 (2014).
Mougel, C. et al. Dynamic of the genetic structure of bacterial and fungal communities at different developmental stages of Medicago truncatula Gaertn. cv. Jemalong line J5. New Phytol. 170, 165–175 (2006).
Berg, G. & Smalla, K. Plant species and soil type cooperatively shape the structure and function of microbial communities in the rhizosphere. FEMS Microbiol. Ecol. 68, 1–13 (2009).
Chaparro, J. M. et al. Exudation of phytochemicals in Arabidopsis follows specific patterns that are developmentally programmed and correlate with soil microbial functions. PLoS One 8(2), 1–10 (2013).
Marques, J. M. et al. Plant age and genotype affect the bacterial community composition in the tuber rhizosphere of field-grown sweet potato plants. FEMS Microbiol. Ecol. 88, 424–35 (2014).
Wagner, M. R. et al. Host genotype and age shape the leaf and root microbiomes of a wild perennial plant. Nature Comm. 7, 12151 (2016).
Jacobsen, C. S. & Hjelmsø, M. H. Agricultural soils, pesticides and microbial activity. Curr. Opin. Biotechnol. 27, 15–20 (2014).
Nettles, R. et al. Influence of pesticide seed treatments on rhizosphere fungal and bacterial communities and leaf fungal endophyte communities in maize and soybean. Appl. Soil Ecol. 102, 61–69 (2016).
Likar, M., Stres, B., Rusjan, D., Potisek, M. & Regvar, M. Ecological and conventional viticulture gives rise to distinct fungal and bacterial microbial communities in vineyard soils. Appl. Soil Ecol. 113, 86–95 (2017).
Dini-Andreote, F., Nunes da Rocha, U., Araùjo, W. L., Azevedo, J. L. & Van Overbeek, L. S. Effect of bacterial inoculation, plant genotype and developmental stage on root-associated and endophytic bacterial communities in potato (Solanum tuberosum). Ant. Leeuwen. Internat. J. Gen. Molec. Microbiol. 97, 389–399 (2010).
Sasse, J., Martinoia, E. & Northen, T. Feed your friends: do plant exudates shape the root microbiome? Trends Plant Sci. 23, 25–41 (2018).
O’Donnell, A. G., Seasman, M., Macrae, A., Waite, I. & Davies, J. T. Plants and fertilizers as drivers of change in microbial community structure and function in soils. Plant Soil 232, 135–145 (2001).
Muñoz-Leoz, B., Garbisu, C., Antigüedad, I. & Ruiz-Romera, E. Fertilization can modify thenon-target effects of pesticides on soil microbial communities. Soil Biol. Biochem. 48, 125–134 (2012).
Mijangos, I., Pérez, R., Albizu, I. & Garbisu, C. Effects of fertilization and tillage on soil biological parameters. Enz. Micr. Technol. 40, 100–106 (2006).
Černohlávková, J. Effects of selected environmental pollutants on soil microbial community in laboratory and field studies. Dissertation Thesis, RECETOX—Research Centre for Environmental Chemistry and Ecotoxicology, Brno, Czech Republic (2009).
Franklin, R. B. & Mills, A. L. Multi-scale variation in spatial heterogeneity for microbial community structure in an eastern Virginia agricultural field. FEMS Microbiol. Ecol. 44, 335–346 (2003).
Nunan, N., Wu, K., Young, I. M., Crawford, J. W. & Ritz, K. Spatial distribution of bacterial communities and their relationship with the micro-architecture of soil. FEMS Microbiol. Ecol. 44, 203–215 (2003).
Tecon, R. & Or, D. Biophysical processes supporting the diversity of microbial life in soil. FEMS Microbial. Rev. 41, 599–623 (2017).
Karagöz, K., Ates, F., Karagöz, H., Kotan, R. & Çakmakçi, R. Characterization of plant growth-promoting traits of bacteria isolated from the rhizosphere of grapevine grown in alkaline and acidic soils. Eur. J. Soil Biol. 50, 144–150 (2012).
Campisano, A. et al. Bacterial endophytic communities in the grapevine depend on pest management. PLoS One 9, e02527–14 (2014).
Bulgari, D., Casati, P., Quaglino, F. & Bianco, P. A. Endophytic bacterial community of grapevine leaves influenced by sampling date and phytoplasma infection process. BMC Microbiol. 14, 198 (2014).
Vega-Avila, A. D. et al. Bacterial communities in the rhizosphere of Vitis vinifera L. cultivated under distinct agricultural practices in Argentina. Ant. Van Leeuwen. Internat. J. Gen. Mol. Microbiol. 107, 575–588 (2015).
Ahn., J. H. et al. Characterization of the bacterial and archaeal communities in rice field soils subjected to long-term fertilization practices. J. Microbiol. 50, 754–765 (2012).
Jenkins, S. N. et al. Actinobacterial community dynamics in long term managed grasslands. Ant. van Leeuwen. Internat. J. Gen. Mol. Microbiol. 95, 319–334 (2009).
Daquiado, A. R. et al. Pyrosequencing analysis of bacterial community diversity in long-term fertilized paddy field soil. Appl. Soil Ecol. 108, 84–91 (2016).
Lesaulnier, C. et al. Elevated atmospheric CO2 affects soil microbial diversity associated with trembling aspen. Environ. Microbiol. 10, 926–941 (2008).
Berendesen., R. L., Pieterse, C. M. J. & Bakker, P. A. H. M. The rhizosphere microbiome and plant health. Trends Plant Sci. 17, 478–486 (2012).
Philippot, L., Raaijmakers, J., Lemanceau, P. & van der Putten, W. H. Going back to the roots: the microbial ecology of the rhizosphere. Nat. Rev. Microbiol. 11, 789–799 (2013).
Albuquerque, L. et al. Gaiella occulta gen. nov., sp. nov., a novel representative of a deep branching phylogenetic lineage within the class Actinobacteria and proposal of Gaiellaceae fam. nov. and Gaiellales ord. nov. Syst. Appl. Microbiol. 34, 595–599 (2011).
Baudoin, E., Benizri, E. & Guckert, A. Impact of growth stage on the bacterial community structure along maize roots, as determined by metabolic and genetic fingerprinting. Appl. Soil Ecol. 19, 135–145 (2002).
Houlden, A., Timms-Wilson, T. M., Day, M. J. & Bailey, M. J. Influence of plant developmental stage on microbial community structure and activity in the rhizosphere of three field crops. FEMS Microbiol. Ecol 65, 193–201 (2008).
Micallef, S. A., Channer, S., Shiaris, M. P. & Colón-Carmona, A. Plant age and genotype impact the progression of bacterial community succession in the Arabidopsis rhizosphere. Plant Signal. Behav. 4, 777–780 (2009).
Gregory, P. J. Roots, rhizosphere and soil: The route to a better understanding of soil science? Eur. J. Soil Sci. 57, 2–12 (2006).
Newman, M. M. et al. Glyphosate effects on soil rhizosphere-associated bacterial communities. Sci. Tot. Environ. 543, 155–160 (2016).
Donn, S., Kirkegaard, J. A., Perera, G., Richardson, A. E. & Watt, M. Evolution of bacterial communities in the wheat crop rhizosphere. Environ. Microbiol. 17, 610–621 (2015).
Barea, J. M., Pozo, M. J., Azcón, R. & Azcón-Aguilar, C. Microbial co-operation in the rhizosphere. J. Exp. Bot. 56, 1761–1778 (2005).
Parke, J. L. & Gurian-Sherman, D. Diversity of the Burkholderia cepacia complex and implications for risk assessment of biological control strains. Ann. Rev. Phytopathol. 39, 225–258 (2001).
Perrin, E. et al. Exploring the HME and HAE1 efflux systems in the genus Burkholderia. BMC Evolut. Biol. 10, 164 (2010).
Krechel, A., Faupel, A., Hallmann, J., Ulrich, A. & Berg, G. Potato-associated bacteria and their antagonistic potential towards plant-pathogenic fungi and the plant-parasitic nematode Meloidogyne incognita (Kofoid & White) Chitwwod. Can. J. Microbiol. 48, 772–786 (2002).
Young, C. & Otto, M. Staphylococcus epidermidis infections. Micr. Infect. 4, 481–489 (2002).
Takeuchi, F. et al. Whole-genome sequencing of Staphylococcus haemolyrticus uncovers the extreme plasticity of its genome and the evolution of human-colonizing staphylococcal species. J. Bacteriol. 187, 7292–7308 (2005).
Tyler, H. L. & Triplett, E. W. Plants as a habitat for beneficial and/or human pathogenic bacteria. Ann. Rev. Phytopathol. 46, 53–73 (2008).
Berg, G. et al. Plant-dependent genotypic and phenotypic diversity of antagonistic rhizobacteria isolated from different Verticillium host plants. Appl. Environ. Microbiol. 68, 3328–3338 (2002).
Berg, G., Eberl, L. & Hartmann, A. The rhizosphere as a reservoir for opportunistic human pathogenic bacteria. Environ. Microbiol. 7, 1673–1685 (2005).
Berg, G., Erlacher, A., Smalla, K. & Krause, R. Vegetable microbiomes: is there a connection among opportunistic infections, human health and our ‘gut feeling’? Micr. Biotechnol. 7, 487–495 (2014).
Gupta, C. P., Sharma, A., Dubey, R. C. & Maheshwari, D. K. Effect of metal ions on growth of Pseudomonas aeruginosa and siderophores and protein function. J. Exp. Biol. 39, 1318–1321 (2001).
Mehnaz, S. et al. Isolation and 16S rRNA sequence analysis of the beneficial bacteria from the rhizosphere of rice. Can. J. Microbiol. 47, 110–117 (2001).
Sessitsch, A., Reiter, B. & Berg, G. Endophytic bacterial communities of field-grown potato lines and their plant growth-promoting abilities. Can. J. Microbiol. 50, 239–249 (2004).
Yousaf, S. et al. Pyrosequencing detects human and animal pathogenic taxa in the grapevine endosphere. Front. Microbiol. 5, 237 (2014).
Campisano, A. et al. Interkingdom transfer of the acne-causing agent, Propionibacterium acnes, from human to grapevine. Mol. Biol. Evol. 31, 1059–1065 (2014).
Weisburg, W. G., Barns, S. M., Pelletier, D. A. & Lane, D. J. 16S ribosomal DNA amplification for phylogenetic study. J. Bacteriol. 173, 697–703 (1991).
Wang, Y. & Qian, P. Y. Conservative fragments in bacterial 16S rRNA genes and primer design for 16S ribosomal DNA amplicons in metagenomics studies. PLoS ONE 4, e7401 (2009).
Ewing, B., Hillier, L., Wendl, M. & Green, P. Base-calling of automated sequencer traces using phred. I. Accuracy assessment. Genome Res. 8, 175–185 (1998).
Wang, Q., Garrity, G. M., Tiedje, J. M. & Cole, J. R. Naive Bayesian classifier for rapid assignment of rRNA sequences into the new bacterial taxonomy. Appl. Environ. Microbiol. 73, 5261–5267 (2007).
R Core Team. R: A language and environment for statistical computing. R Foundation for Statistical Computing, Vienna, Austria. (2018).
Dhariwal, A. et al. MicrobiomeAnalyst – a web-based tool for comprehensive statistical, visual and meta-analysis of microbiome data. Nucl. Acids Res. 45, W180–188, https://doi.org/10.1093/nar/gkx295 (2017).
Source: Ecology - nature.com