Significant intensification of Sahara dust intrusions has been noticed during the last few decades50. These intrusions, and the subsequent dust deposition, are relevant for nutrient dispersal and soil development51,52, as well as for the introduction and dispersal of external microorganisms, including pathogens, into ecosystems and populations17,30,53. Despite the relevance of these phenomena, there are few studies analyzing variations on the atmospheric microbial diversity as a result of Sahara dust intrusions in Southern Europe34,35,54,55. Here, we analyzed the microbial load of one of the largest winter-occurring Saharan dust events in the Iberian Peninsula and, for the first time, we offer new insights into bacterial distribution at different altitudes of the lower troposphere, as well as the replacement of the native microbial airborne structure as the dust event disappears.
Samples collected and microscopic analysis
The sampling campaign operated three continental flights within the Iberian Peninsula (flights from Madrid to Avila and Lugo (NW Spain)) and one flight off the coast in the north (Fig. 1) during which samples were taken. Macroscopic differences among the filters in terms of dust/particles recovery were clearly distinguished, showing lower coloration as the altitude increases (Suplementary Fig. S1). SEM analysis showed that the samples collected during the dust event were a collection of loose grains to which small particles adhered (Suplementary Fig. S2). Under these in situ conditions, it was difficult to distinguish microbes from clay particles, diatom fragments and other adhering material. As expected, samples from the other flights showed lower amounts of dust particles. Nevertheless, obvious cellular structures were visible in all samples analyzed. The most frequent images clearly shows rounded individual cells-like particles of ca. 6–10 μm, as well as cells forming clusters of variable shapes and sizes. Cells and clusters are always covered by an irregular coat of extracellular polymeric substances (EPSs). Fragments of diatom shells could be also seen.
Dust particles showed irregular morphology and great size variability (<10–30 μm) (Suplementary Fig. S2A, B). Elemental composition determination by SEM-EDS analysis showed that particles collected during the Saharan intrusion were composed mainly of Si (ca. 50 Wt%), Al and Fe (ca. 10 Wt%), as well as some trace elements such as Ti, Cr, Pb, Sr, Ba, Zr, Ag and Mn (Suplementary Fig. S3). These trace elements were not found in the remaining samples. Particles from flights F28, F9 and F10, were mainly composed by NaCl, although F28 presented a lesser degree of ions related to salt, and a major influence from soil.
Air mass back trajectories pointed to the Saharan/Hoggar Massif area as the main origin of this dust episode. In this region, the local geology is dominated by carbonate depocenter56. Soils from this desert area show high chemical diversity (i.e. hornblende and alkali feldspar, kaolinite, illite or montmorillonite)56. The results of EDS analysis showed a higher presence of Si, Mg, K, Mn and some trace elements such as Ti, V, Sr, Ba, Zr, Ni, Cr, Mo, Cu, Ag and Pb during Saharan dust intrusion. Average concentrations during non-Saharan dust periods (Atlantic air mass), showed higher amounts of Fe, Al, Na and Cl and none of the trace elements present during the dust event.
The major elements identified in the filters during the dust event showed the representative composition of particulate matter from the Saharan desert57. However, trace element composition present in the samples are not characteristic of the area and could be related to industrial emissions, Thus, the main sources of Ti, V, Mo, Cr and Pb are oil refineries, power plants, and phosphate-based fertilizer plants located in North Africa and Southern Spain58. Therefore, given the direction of the air masses and the composition of the particulate matter in the samples during the dust event, we suggest that the dust collected there came mainly from the Sahara, although some additional industrial emission material was collected on its way from the Sahara to Spain. This fact has also been reported by Sánchez de la Campa et al. (2013) in the only microbiological study related to Sahara dust intrusions in Spain conducted to date.
Trayectory analysis
Dust concentration from 23 February to 10 March 2017, in 2-day intervals is summarized in Fig. 3. As observed, this period was characterized by a Saharan dust intrusion in the Iberian Peninsula, which was particularly strong between 23–24 February. After this date, the wind pattern above the Iberian Peninsula had changed to predominant north-northwesterly winds coupled with precipitation during these days, that favored dust deposition in the Iberian Peninsula (see the dust concentration decrease after 24 February in Fig. 3).
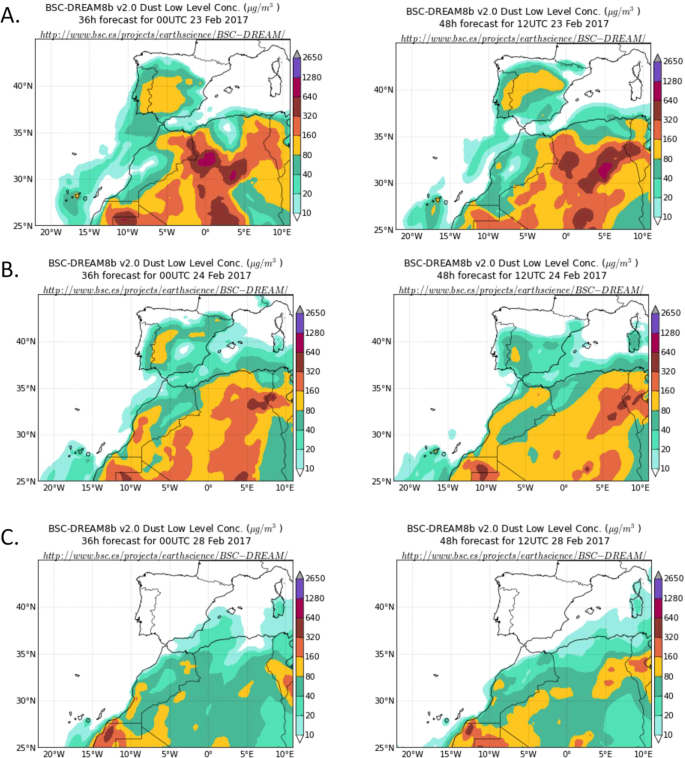
Dust concentration (μg/m3) predicted by model BSC-DREAM8b v2.0 for (A) 23 February 2017 at 00 UTC (left) and 12 UTC (right). (B) 24 February 2017 at 00 UTC (left) and 12 UTC (right). (C) 28 February 2017 at 00 UTC (left) and 12 UTC (right). On March 9th and 10th, dust concentration data for the Iberian Peninsula was very similar to that of February 28th (data not shown), predicted by model BSC-DREAM8b v2.0, Barcelona Supercomputing Center.
Air masses sampled on each flight were found to have a different origin on average, based on HYSPLIT simulations. F24-HT, sampled on Feb 24 at ~3,000 m, showed air masses coming both from North Africa and the Atlantic Ocean (Fig. 4A). Thus, those coming from North Africa (Feb 22–23) experienced strong vertical mixing with different atmospheric layers, including the Saharan Planetary boundary layer (PBL)33,59. On Feb 24, the wind pattern changed above the Iberian Peninsula, rotating to north-northwesterly winds; as a result, the origin of air masses was originated in the Atlantic Ocean for this particular day. Therefore, the resulting Saharan dust remaining in the atmosphere is expected to be the main source contributing to the sample. F24-LT, roughly sampled at the same time and location as F24-HT, but at ~100 m above the ground level (Suplementary Table S1), showed a similar change in the air mass pattern. On Feb 22 and Feb 23 predominant air masses originated from the eastern part of the Iberian Peninsula and Spain’s Mediterranean coast, with strong interaction within the PBL above the southeast and center of the Iberian Peninsula. On the contrary, predominant air masses on Feb 24 came from the north of Iberian Peninsula. As a result, this sample was influenced by the Iberian Peninsula’s PBL, in addition to the large amount of Saharan dust present at this time (Fig. 4B) which was previously transported by air masses at higher altitudes33.
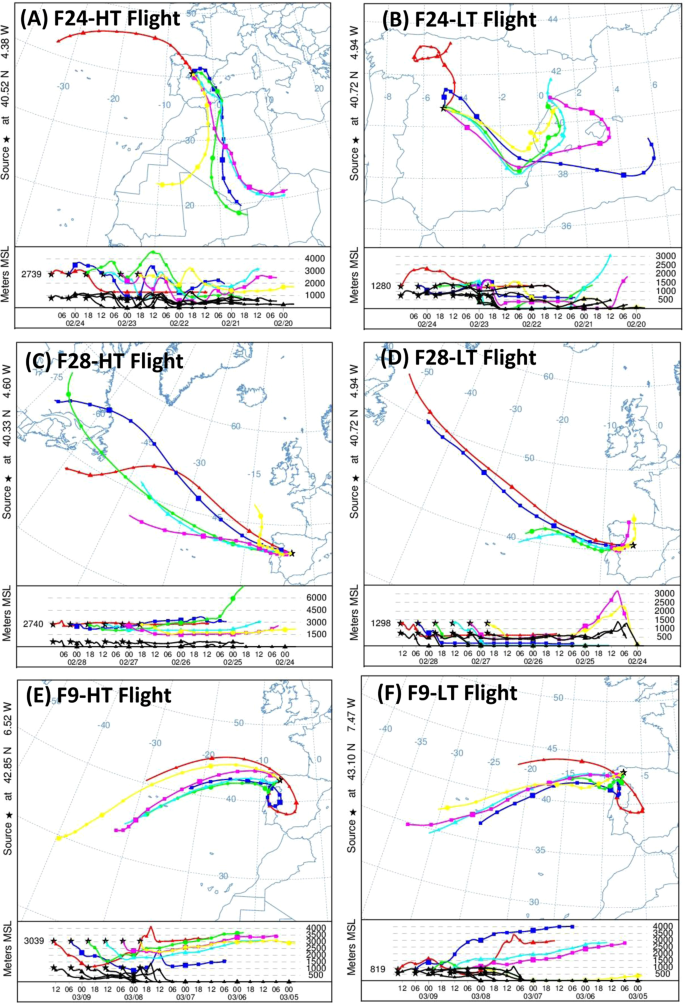
Three-days back-trajectories computed by HYSPLIT for the different sampling area flights. Air mass trajectories within the 48h-interval previous to the sampling time. (A) F24-HT flight (3,000 m altitude); (B) F24-LT flight (100 m altitude); (C) F28-HT flight (3,000 m altitude); (D) F28-LT flight (100 m altitude); (E) F9-HT flight (3,000 m altitude); (F) F9-LT flight (100 m altitude). F10 flights presented similar trajectories than F9 flights.
The situation was completely different in the case of F28-HT (Fig. 4C). During this flight, samples were obtained at ~3,000 m, as in F24-HT but, as previously described, dust intrusion had finalized. Wind pattern above the Iberian Peninsula changed to predominant north-northwesterly winds, and precipitation favored dust settling on the Iberian Peninsula. Thus, back-trajectories computed via HYSPLIT showed predominant westerly air masses originating (72-hours) from the free troposphere above the Atlantic Ocean. A similar pattern was roughly observed in F28-LT (Fig. 4D), where the air masses also interacted with the Iberian Peninsula and marine PBL’s.
F9-HT back-trajectories showed predominant air masses originating from the Atlantic Ocean (72 h), after strong recirculation above the northwest side of the Iberian Peninsula (Fig. 4E), in addition to a direct interaction with lower atmospheric layers, including the PBL in some cases, due to the vertical movement of the air masses. This recirculation was also observed in F9-LT, although to a lesser extent, taking into account the transport of the air masses through the PBL (Fig. 4F). Back-trajectories for F10-LT and F10-HT are not shown here because they were similar to those presented for F9-LT and F9-HT, respectively. The summary of back-trajectory results as well as the predominant source for every sample is shown in Suplementary Table S1.
Sequencing analysis and estimated richness
The collections of amplicons were sequenced, and the number of reads is summarized in Supplementary Table S2. After total DNA extraction from each of the collected samples, 16 S rDNA PCR amplification and DNA sequencing, a total of ∼1.5 ×106 sequences were obtained across all samples, about 120,000 per sample on average. The analysis of the read length distributions indicated a modal distribution between 442 and 444 nt. After quality control checks, chimera sequence removal and controls sequences subtraction, a total of ∼140,000 sequences remained, about 11,000 per sample on average. Chloroplast sequences were detected (and removed) in all samples, with their proportion varying between 0.47 and 5.2%. The number of OTUs detected at a distance of 0.03, was between 30 to 375 per sample, with a total of 1,708 of bacterial OTUs observed. Nevertheless, Chao1estimations suggest that we were able to identify from 72 to 96% of the total OTU population. In general, samples taken during the flights showed lower number of OTUs than the surface samples (∼50% less).
Samples were collected at different altitudes (Surface, 100 m and 3,000 m) during three different atmospheric scenarios, a severe winter Saharan dust intrusion (23–24 February), a dust free environment after intense rains (28 February) and two weeks after the dust event (9–10 March). It is interesting to note that during dust event days, samples from the surface and flights showed a higher richness in bacterial population (Chao1) than the samples from non-dust event days. Conversely, although Shannon H diversity index showed higher values in the surface samples, similar values were found between dust-event days and non-dust samples. However, the Shannon H index was generally higher in surface samples than in the flight ones (∼47% less), except during the dust-event, in which no differences were found between surface and flight samples. Besides, sample F28-LT showed the lowest diversity (Shannon H index 0.5). Although similar studies of airborne bacterial diversity across different altitudes during a dust intrusion are currently nonexistent, our results are comparable to the diversity patterns observed in other studies dealing with desert dust events. Thus, samples collected during the dust intrusion showed a higher diversity (Chao1 and Shannon H index, Supplementary Table S2) than non-dust related samples, which is in agreement with other previous studies4,13,30,34,35,60. Additionally, rarefaction analysis (Suplementary Fig. S4) revealed that tropospheric communities are less complex compared to many habitats on Earth such as soils or waters61.
Diversity of bacteria
Sequences were affiliated with 27 bacterial phyla and/or classes, among which Alphaproteobacteria (∼60%), Actinobacteria (∼10%), Firmicutes (∼10%) and Bacteroidetes (∼7%) were dominant, representing ∼90% of the total microbial community (Fig. 5, Suplementary Table S3). Approximately 7% of representative sequences could not be classified. Only six phyla were detected exclusively in the samples collected during the dust-event days, Armatimonadetes, Candidate division WPS- 1 and 2, Nitrospirae, Parcubacteria and Synergistetes, although each of them accounted for less than 1% of the sequences. In order to reveal any underlying trends in the composition of the different bacterial populations, hierarchical dendrogram at the phylum/class level (Suplementary Fig. S5) was generated via the Euclidean squared distance method. All the samples associated with dust intrusion (SU-23F, SU-24F, F24-HT and F24-LT) clustered together, and very near to the surface samples collected during the non-dust event (SU-28F and SU-9M). Additionally, samples from flights after the rain episode (F28-HT and F28-LT) grouped together, showing also the highest amounts of Alphaproteobaterias in F28-LT samples (∼90% of the total sequences) and Chloroflexi in F28-HT (∼9% of the total sequences). On the other hand, low troposphere flight samples from non-dust event day (F9-LT, F9-HT) as well as samples taken during the off-shore flights (F10-LT and F10-HT) showed a completely different microbial composition and clustered together. Samples from flight F9-LT revealed the highest amounts of Fusobacteria (96% of the total Fusobacteria related sequences) and Cloacimonetes were detected only in samples from off-shore flights (F10-LT and F10-HT). These samples also produced the highest percentages of Spirochaetes and Saccharibacteria (94% and 75% of the total respective phylum sequences).
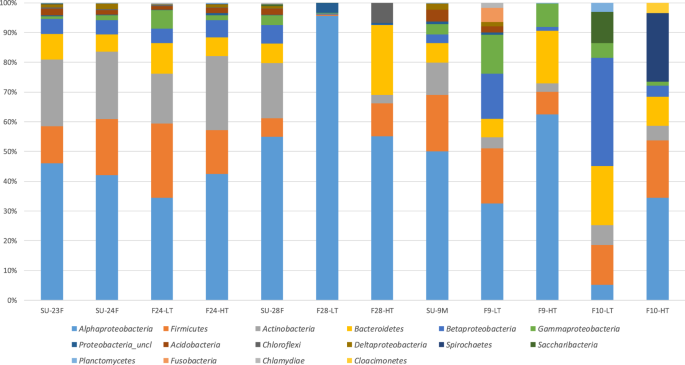
Relative abundance and Phylum-class level distribution of bacterial OTUs for each sampling. Only groups with relative abundances >1% are shown. Classification and histogram were performed by Mothur package.
In turn, SSU rRNA gene sequences Principal Component Analysis (PCA) at 0.03 distance (Fig. 6), as well as the associated hierarchical clustering (Suplementary Fig. S6) was performed, explaining 71% of the total variance with three components. The PCA analysis revealed three major clusters among the samples, revealing that samples related in height or collection time seem to show more similar community composition patterns when compared to unrelated samples. The first cluster grouped all the surface samples (SU-23F, SU-24F, SU-28F and SU-9M) plus the samples from the first flight collected during the dust-event (F24-LT and F24-HT). This results reflected the influence of the dust intrusion in the microbial composition of the low troposphere, suggesting that desert dust intrusions aerosolize a large number of new cells and taxa, dramatically affecting the composition of the low tropospheric microbial communities, at least up to the 3,000 m sampled. Back trajectory analysis (22–23 Feb) showed that air mases coming from North Africa (and microbial cells transported with them) experienced a strong vertical mixing with different atmospheric layers, including the Saharan Planetary boundary layer (PBL) in origin, and were brought aloft during the dust intrusion event. This could explain the similarity among the samples taken at different altitudes during the intrusion.
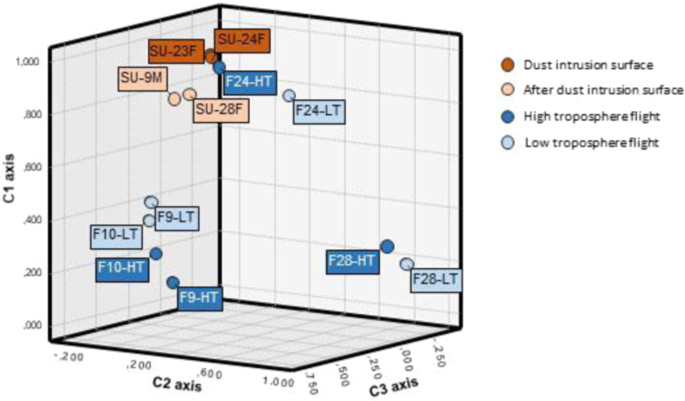
Principal Component Analyses (PCA) on the relative proportion of OTUs (at a 0.03 distance).
Interestingly, surface samples from days after the dust intrusion event (SU-28F and SU-9M) are also included in this cluster (although forming a subcluster), showing a clear similarity with the microbial community during the dust intrusion (Fig. 6). This result suggest that, changes in the local atmospheric microbial composition near the surface caused by the dust intrusions may remain for several days after the major event has passed60. This desert influence in the atmosphere could extend even to several weeks in the presence of thermal inversion phenomena, which are frequently associated with hot air desert intrusions in the Iberian Peninsula during the winter62.
The Saharan origin of these microbial communities is revealed by the high conformity of principal bacterial genera described for Saharan desert with those present in these samples63. Thus, the most abundant bacterial species in all samples taken during the dust event (∼30–40% of the total sequences in these samples) (Suplementary Table S3), gruped in three different phyla, previously reported during desert dust events35,60,63,64,65: (a) Proteobacteria–Rhizobiales (Rhizobium and Microvirga), Sphingomonadales, Rhodobacterales (Rubellimicrobium), (b) Actinobacteria– Geodermatophilaceae; (c) Firmicutes– Bacillaceae,. Most of these species are well known for being extremely stress-resistant, including, high temperatures, such as Rubellimicrobium63, high radiation and desiccation, specially Geodermatophilus64,65, or spore formation such as Bacillus, which increase the probability of survival during transportation66.
Additionally, other highly stress-resistant bacteria groups were also detected, although in low amounts, representing always less than 1% of the total sequences in each sample. Thus, species related to, (a) high temperature: Pseudonocardia, Streptomyces, Thermomonosporaceae, Nitrospira and Porphyrobacter; (b) radiation: Deinococcus, Hymenobacter, Methylobacterium and Rubrobacter; (c) desiccation: Kineococcus and Methylobacterium; (d) psychrotolerance: Planococcus, Subtercola and Modestobacter; and (e) halophyly: Salinarimonas and Nocardioides, were also detected in all the samples related to the dust event (SU-23F, SU-24F, F24-LT, F24-HT) as well as in the surface samples taken after it (SU-28F, SU-9M).
The second cluster observed in the PCA analysis (Fig. 6 and Suplementary Fig. S6) grouped the samples obtained during the second flight, after the rain event (F28-LH and F28-HT), pointing out a completely different situation. After the rain, the atmospheric microbial community from samples collected during the flights changed completely, with samples being clearly related by time scale rather than altitude. Thus, samples from flights after the rain period (F28-LT and F28-HT) clustered together and separately from the samples taken during the flights two weeks after the dust event (F9-LT, F9-HT, F10-LT and F10-HT). These results reflected the completely different atmospheric situation once the dust intrusion was over. Winds pattern above the Iberian Peninsula changed to predominant north-northwesterly winds, and the precipitations favored dust deposition. Our results are in agreement with previous studies in which it has been hypothesized that an important variable affecting the differences in atmospheric bacterial composition may be due to variability in the predominant winds at any given time64,65. In these samples, Rhizobium is the main represented genera (∼40–90% of the total sequences in these samples). Rhizobium is a sporulating Gram-negative soil bacteria that form endosymbiotic nitrogen-fixing associations with plant roots such as legumes67. Consistent analysis from previous studies reported that, in continental and temperate regions, plants are one of the main sources of atmospheric bacteria. This is suggested by the large quantity of plant associated bacteria, such as Sphingomonadales and Rhizobiales, and the high number of chloroplast sequences retrieved in the samples collected (data not shown). Besides, a previous study demonstrated that soil-inhabiting bacteria, such as Actinobacteriales, Firmicutes and Rhizobiales, prevailed in the atmosphere during cold seasons68. In our case, it is tentative to suggest that the abundance of Rhizobiales in the atmosphere could be a result of the land area surrounding the flight samplings, which are mainly used for agricultural purposes, where seasonal plowing and cultivation processes may contribute to the atmospheric bacterial sources69. Rhizobiales were also found in the dust event related samples, but represented less than the 5% of the total sequences found.
PCA analysis also revealed that the microbial communities in the samples taken during the flights that took place 15 days after the dust event (F9-HT, F9-LT, F10-HT and F10-LT) were much more similar among them when compared to the other samples collected. In this case, Brevundimonas, and Methylobacterium as well as Cupriavidus and Mesorizobium were the most abundant genera respectively (∼20–50% of the total sequences in these samples) (Fig. 6 and Suplementary Fig. S6). Brevundimonas species are ubiquitous in the environment and is one of few bacteria showing high survival rates under radiation simulating Martian conditions, while some species of Cupriavidus are known by their high resistance to oxidative stress70,71.
Our results showed that, despite the similitudes in the bacterial diversity between our atmospheric samples and the previously reported ones, there are a number of important and stark differences. For example, we did not find any sequence related to Afipia sp., one of the main constituents of the troposphere community (>40% of total community) reported by DeLeon-Rodriguez et al. (2013), as well as some of the most abundant families recently described by Smith et al. (2018) in the lower stratosphere (such as Staphylococcaceae, Moraxellaceae, Lachnospiraceae and Ruminococcaceae), although we found a similar number of OTUS. Furthermore, one of the main components found in our samples related to Saharan intrusion, Microvirga sp., has not been reported previously in the consulted literature. Additionally, contrary to previous studies, we did not find what has been described as a “core microbiome”36 since there are no bacterial species common to all the samples analyzed, not even within the flight samples.
On the other hand, Triado-Margarit et al. (2019) reported that the bacterial composition of bioaerosols collected by passive natural deposition at high-elevated mountains were closer to the bacterial microbiome from the free troposphere. Our results showed also comparable patterns, since samples from the same flights at low and high troposphere were similar in terms of bacterial diversity. Besides, our analysis showed a different bacterial community structure in samples collected over the open ocean, during desert dust events or after a rain period, as described in the mentioned study72. Interestingly, these authors stated that only Oxalobacteraceae were notably more abundant in wet deposition, with Noviherbaspirillum and Massilia as dominant genera72. In our case, although these genera have been found in most of the samples, they account for less than 1% of the total community in all cases. These examples support the idea of a highly dynamic and diverse atmospheric microbiome that deserve considerably more attention due to the different bioaerosol origins, the assortment of known emission sources and the potential for long range atmospheric dispersal31.
Potential health threats
After long-range transport, the diversity of bacteria associated with dust events may play a significant role in human, plant and animal health, although it is not clear what are the limits on the overall transport of pathogens around the planet. We have found that most of the potential pathohogenic-relevant bacteria are mainly found in the dust event related samples, in both surface and flight samples. Although HtS methods did not permit assigning species, several environmental genera that contain opportunistic pathogens25,27,73, were identified including Legionella, Neisseria, Staphylococcus, Chlamydia, Acinetobacter, Arthrobacter, Gordonia, Kocuria, Brevibacterium, Rasltonia, Pantoea, Pseudomonas, Mycobacterium and Bacillus.
Special attention should be paid to the samples taken during the flights carried out on 9 March, in which approximately 50% of the sequences corresponded to the genus Brevundimonas, a Gram-negative bacterium recently described as an emerging global opportunistic pathogen in nosocomial infections72. Furthermore, sequences related to Brucella (associated to brucellosis) and Haemophilus (associated to bacterial flu) genera were also found in significant amounts in these flight samples. Although no reports of human infectious diseases related to long-distance dispersal of pathogens associated to dust events exists to date, aside from increasing incidences of asthma, the World Health Organization has reported dust storm events in the sub-Sahara region as one of the main origins for local occurrences of meningococcal meningitis (Neisseria meningitidis), causing around 50,000 deaths every year73. Thus, enhanced desertification will introduce more dust into the atmosphere, increasing the importance of monitoring microbial communities in intercontinental winds. This is especially relevant in terms of the aerial dispersal of plant pathogens, due to the limited genetic diversity of the majority of the modern crops that make them highly susceptible to new pathogens17,20.
Overall, we observed that samples related in height or time scale showed more similar community composition patterns compared with unrelated samples. Additionally, the dust intrusion had a profound influence in the microbial composition of free trophosphere areas with little influence of ground surface contamination, such as areas located up to 3,000 m. In this study, Sahara desert storms were able to aerosolize a large number of cells and species that have been distributed along the entire air column, strongly resembled the bioaerosols collected near the ground even 15 days after the dust event. There is still a lack of knowledge about the atmospheric microbial community, its spatial and temporal distribution, how they can adapt to this environment and wheter they are able to actively metabolize organic and inorganic compounds and proliferate in order to consider the atmosphere as an ecosystem in its own right rather than as a conduit for life. It is also important to understand how atmospheric phenomena (i.e. dust storms, hurricanes, etc…) suspend and transport microbial communities globally. For this reason, there is a need for improvement and implementation of suitable sampling systems and the development of manned and unmanned aerial systems capable of sampling large volumes of air for subsequent analyses, as it would provide a wide range of applications in atmospheric, environmental and health sciences.
Source: Ecology - nature.com