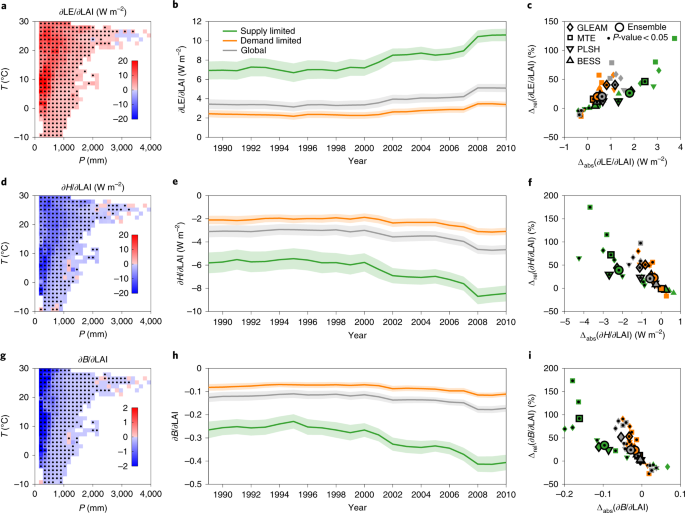
Findell, K. L., Gentine, P., Lintner, B. R. & Kerr, C. Probability of afternoon precipitation in eastern United States and Mexico enhanced by high evaporation. Nat. Geosci. 4, 434–439 (2011).
Pielke, R. A. Influence of the spatial distribution of vegetation and soils on the prediction of cumulus convective rainfall. Rev. Geophys. 39, 151–177 (2001).
Pitman, A. J. The evolution of, and revolution in, land surface schemes designed for climate models. Int. J. Climatol. 23, 479–510 (2003).
Seneviratne, S. I. et al. Investigating soil moisture–climate interactions in a changing climate: a review. Earth Sci. Rev. 99, 125–161 (2010).
Dirmeyer, P. A. The terrestrial segment of soil moisture–climate coupling. Geophys. Res. Lett. 38, L16702 (2011).
Puma, M. J., Koster, R. D. & Cook, B. I. Phenological versus meteorological controls on land–atmosphere water and carbon fluxes. J. Geophys. Res. Biogeosci. 118, 14–29 (2013).
Williams, I. N. & Torn, M. S. Vegetation controls on surface heat flux partitioning, and land–atmosphere coupling. Geophys. Res. Lett. 42, 9416–9424 (2015).
Bagley, J. E. et al. The influence of land cover on surface energy partitioning and evaporative fraction regimes in the U.S. Southern Great Plains. J. Geophys. Res. Atmos. 122, 5793–5807 (2017).
Zhu, Z. et al. Greening of the Earth and its drivers. Nat. Clim. Change 6, 791–795 (2016).
Anderson, R. G. et al. Biophysical considerations in forestry for climate protection. Front. Ecol. Environ. 9, 174–182 (2011).
Bonan, G. B. Forests and climate change: forcings, feedbacks, and the climate benefits of forests. Science 320, 1444–1449 (2008).
Forzieri, G., Alkama, R., Miralles, D. G. & Cescatti, A. Satellites reveal contrasting responses of regional climate to the widespread greening of Earth. Science 356, 1180–1184 (2017).
Pitman, A. J. et al. Importance of background climate in determining impact of land-cover change on regional climate. Nat. Clim. Change 1, 472–475 (2011).
Berry, J. A., Beerling, D. J. & Franks, P. J. Stomata: key players in the earth system, past and present. Curr. Opin. Plant Biol. 13, 232–239 (2010).
Keenan, T. F. et al. Increase in forest water-use efficiency as atmospheric carbon dioxide concentrations rise. Nature 499, 324–327 (2013).
Zeng, Z. et al. Climate mitigation from vegetation biophysical feedbacks during the past three decades. Nat. Clim. Change 7, 432–436 (2017).
Wilson, K. B. et al. Energy partitioning between latent and sensible heat flux during the warm season at FLUXNET sites. Water Resour. Res. 38, 1294 (2002).
Sellers, P. J. et al. BOREAS in 1997: experiment overview, scientific results, and future directions. J. Geophys. Res. Atmos. 102, 28731–28769 (1997).
Baldocchi, D. et al. FLUXNET: a new tool to study the temporal and spatial variability of ecosystem-scale carbon dioxide, water vapor, and energy flux densities. Bull. Am. Meteorol. Soc. 82, 2415–2434 (2001).
Piao, S. et al. Evaluation of terrestrial carbon cycle models for their response to climate variability and to CO2 trends. Glob. Change Biol. 19, 2117–2132 (2013).
Duveiller, G. et al. Biophysics and vegetation cover change: a process-based evaluation framework for confronting land surface models with satellite observations. Earth Syst. Sci. Data 10, 1265–1279 (2018).
Forzieri, G. et al. Evaluating the interplay between biophysical processes and leaf area changes in land surface models. J. Adv. Model. Earth Syst. 10, 1102–1126 (2018).
Jiang, C. et al. Inconsistencies of interannual variability and trends in long-term satellite leaf area index products. Glob. Change Biol. 23, 4133–4146 (2017).
Fan, Y., Li, H. & Miguez-Macho, G. Global patterns of groundwater table depth. Science 339, 940–943 (2013).
Morisette, J. T. et al. Tracking the rhythm of the seasons in the face of global change: phenological research in the 21st century. Front. Ecol. Environ. 7, 253–260 (2009).
Miralles, D. G., Gentine, P., Seneviratne, S. I. & Teuling, A. J. Land–atmospheric feedbacks during droughts and heatwaves: state of the science and current challenges. Ann. N. Y. Acad. Sci. 1436, 19–35 (2019).
Zhang, Y. et al. Multi-decadal trends in global terrestrial evapotranspiration and its components. Sci. Rep. 6, 19124 (2016).
Cheng, L. et al. Recent increases in terrestrial carbon uptake at little cost to the water cycle. Nat. Commun. 8, 110 (2017).
Zeng, Z., Peng, L. & Piao, S. Response of terrestrial evapotranspiration to Earth’s greening. Curr. Opin. Environ. Sustain. 33, 9–25 (2018).
Piao, S. et al. Plant phenology and global climate change: current progresses and challenges. Glob. Change Biol. 25, 1922–1940 (2019).
Forzieri, G., Alkama, R., Miralles, D. G. & Cescatti, A. Response to Comment on “Satellites reveal contrasting responses of regional climate to the widespread greening of Earth”. Science 360, eaap9664 (2018).
Mokany, K., Raison, R. J. & Prokushkin, A. S. Critical analysis of root : shoot ratios in terrestrial biomes. Glob. Change Biol. 12, 84–96 (2006).
Chen, C. et al. China and India lead in greening of the world through land-use management. Nat. Sustain. 2, 122–129 (2019).
Le Quéré, C. et al. Global Carbon Budget 2018. Earth Syst. Sci. Data 10, 2141–2194 (2018).
Lian, X. et al. Partitioning global land evapotranspiration using CMIP5 models constrained by observations. Nat. Clim. Change 8, 640–646 (2018).
Buermann, W. et al. Widespread seasonal compensation effects of spring warming on northern plant productivity. Nature 562, 110–114 (2018).
Jiménez, C. et al. Global intercomparison of 12 land surface heat flux estimates. J. Geophys. Res. Atmos. 116, D02102 (2011).
Mahowald, N. et al. Projections of leaf area index in earth system models. Earth Syst. Dynam. 7, 211–229 (2016).
Dai, A. Increasing drought under global warming in observations and models. Nat. Clim. Change 3, 52–58 (2013).
Xiao, Z. et al. Long-time-series global land surface satellite leaf area index product derived from MODIS and AVHRR surface reflectance. IEEE Trans. Geosci. Remote Sens. 54, 5301–5318 (2016).
Zhu, Z. et al. Global data sets of vegetation leaf area index (LAI)3g and fraction of photosynthetically active radiation (FPAR)3g derived from global inventory modeling and mapping studies (GIMMS) normalized difference vegetation index (NDVI3g) for the period 1981 to 2011. Remote Sens. 5, 927–948 (2013).
Claverie, M., Matthews, J. L., Vermote, E. F. & Justice, C. O. A 30+ Year AVHRR LAI and FAPAR Climate data record: algorithm description and validation. Remote Sens. 8, 263 (2016).
Zhang, X., Tan, B. & Yu, Y. Interannual variations and trends in global land surface phenology derived from enhanced vegetation index during 1982–2010. Int. J. Biometeorol. 58, 547–564 (2014).
Kottek, M., Grieser, J., Beck, C., Rudolf, B. & Rubel, F. World map of the Köppen–Geiger climate classification updated. Meteorol. Z. 15, 259–263 (2006).
ESA. Land Cover CCI Product User Guide v.2 (2017).
Miralles, D. G. et al. Global land-surface evaporation estimated from satellite-based observations. Hydrol. Earth Syst. Sci. 15, 453–469 (2011).
Martens, B. et al. GLEAM v3: satellite-based land evaporation and root-zone soil moisture. Geosci. Model Dev. 10, 1903–1925 (2017).
Jung, M. et al. Global patterns of land–atmosphere fluxes of carbon dioxide, latent heat, and sensible heat derived from eddy covariance, satellite, and meteorological observations. J. Geophys. Res. Biogeosci. 116, G00J07 (2011).
Zhang, K. et al. Vegetation greening and climate change promote multidecadal rises of global land evapotranspiration. Sci. Rep. 5, 15956 (2015).
Jiang, C. & Ryu, Y. Multi-scale evaluation of global gross primary productivity and evapotranspiration products derived from Breathing Earth System Simulator (BESS). Remote Sens. Environ. 186, 528–547 (2016).
Dee, D. P. et al. The ERA-Interim reanalysis: configuration and performance of the data assimilation system. Q. J. R. Meteorol. Soc. 137, 553–597 (2011).
Jung, M. et al. Recent decline in the global land evapotranspiration trend due to limited moisture supply. Nature 467, 951–954 (2010).
Siebert, S. et al. A global data set of the extent of irrigated land from 1900 to 2005. Hydrol. Earth Syst. Sci. 19, 1521–1545 (2015).
Pan, F., Peters-Lidard, C. D. & Sale, M. J. An analytical method for predicting surface soil moisture from rainfall observations. Water Resour. Res. 39, 1314 (2003).
Tian, F. et al. Evaluating temporal consistency of long-term global NDVI datasets for trend analysis. Remote Sens. Environ. 163, 326–340 (2015).
Köppen, W. Die Klimate der Erde: Grundriss der Klimakunde (Walter de Gruyter und Co, 1923).
Source: Ecology - nature.com