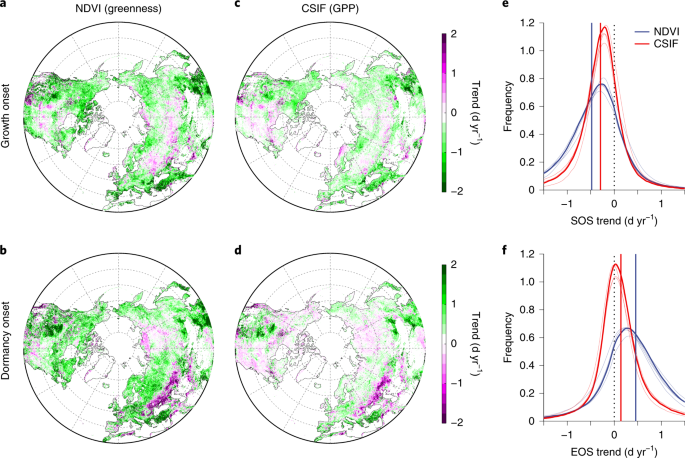
Nemani, R. R. Climate-driven increases in global terrestrial net primary production from 1982 to 1999. Science 300, 1560–1563 (2003).
Zhu, Z. et al. Greening of the Earth and its drivers. Nat. Clim. Change 6, 791–795 (2016).
Jeong, S.-J., Ho, C.-H., Gim, H.-J. & Brown, M. E. Phenology shifts at start vs. end of growing season in temperate vegetation over the Northern Hemisphere for the period 1982–2008. Glob. Change Biol. 17, 2385–2399 (2011).
Buitenwerf, R., Rose, L. & Higgins, S. I. Three decades of multi-dimensional change in global leaf phenology. Nat. Clim. Change 5, 364–368 (2015).
Fridley, J. D., Lynn, J. S., Grime, J. P. & Askew, A. P. Longer growing seasons shift grassland vegetation towards more-productive species. Nat. Clim. Change 6, 865–868 (2016).
Schwartz, M. D., Ahas, R. & Aasa, A. Onset of spring starting earlier across the Northern Hemisphere. Glob. Change Biol. 12, 343–351 (2006).
Richardson, A. D. et al. Climate change, phenology, and phenological control of vegetation feedbacks to the climate system. Agric. For. Meteorol. 169, 156–173 (2013).
Peñuelas, J., Rutishauser, T. & Filella, I. Phenology feedbacks on climate change. Science 324, 887–888 (2009).
Piao, S. et al. Net carbon dioxide losses of northern ecosystems in response to autumn warming. Nature 451, 49–52 (2008).
Keenan, T. F. et al. Net carbon uptake has increased through warming-induced changes in temperate forest phenology. Nat. Clim. Change 4, 598–604 (2014).
Sun, Y. et al. OCO-2 advances photosynthesis observation from space via solar-induced chlorophyll fluorescence. Science 358, eaam5747 (2017).
Guanter, L. et al. Global and time-resolved monitoring of crop photosynthesis with chlorophyll fluorescence. Proc. Natl Acad. Sci. USA 111, E1327–E1333 (2014).
Richardson, A. D. et al. Ecosystem warming extends vegetation activity but heightens vulnerability to cold temperatures. Nature 560, 368–371 (2018).
Ueyama, M., Iwata, H. & Harazono, Y. Autumn warming reduces the CO2 sink of a black spruce forest in interior Alaska based on a nine-year eddy covariance measurement. Glob. Change Biol. 20, 1161–1173 (2014).
Barichivich, J. et al. Large-scale variations in the vegetation growing season and annual cycle of atmospheric CO2 at high northern latitudes from 1950 to 2011. Glob. Change Biol. 19, 3167–3183 (2013).
Zhang, Y., Joiner, J., Alemohammad, S. H., Zhou, S. & Gentine, P. A global spatially contiguous solar-induced fluorescence (CSIF) dataset using neural networks. Biogeosciences 15, 5779–5800 (2018).
Porcar-Castell, A. et al. Linking chlorophyll a fluorescence to photosynthesis for remote sensing applications: mechanisms and challenges. J. Exp. Bot. 65, 4065–4095 (2014).
Krause, G. H. & Weis, E. Chlorophyll fluorescence and photosynthesis: the basics. Annu. Rev. Plant Physiol. Plant Mol. Biol. 42, 313–349 (1991).
Jeong, S. J. et al. Application of satellite solar-induced chlorophyll fluorescence to understanding large-scale variations in vegetation phenology and function over northern high latitude forests. Remote Sens. Environ. 190, 178–187 (2017).
Monteith, J. L. Solar radiation and productivity in tropical ecosystems. J. Appl. Ecol. 9, 747–766 (1972).
Wohlfahrt, G. et al. Trade-offs between global warming and day length on the start of the carbon uptake period in seasonally cold ecosystems. Geophys. Res. Lett. 40, 6136–6142 (2013).
Anav, A. et al. Evaluation of land surface models in reproducing satellite derived leaf area index over the high-latitude northern hemisphere. Part II: Earth system models. Remote Sens. 5, 3637–3661 (2013).
Hu, J., Moore, D. J. P., Burns, S. P. & Monson, R. K. Longer growing seasons lead to less carbon sequestration by a subalpine forest. Glob. Change Biol. 16, 771–783 (2010).
Schuur, E. A. G. et al. The effect of permafrost thaw on old carbon release and net carbon exchange from tundra. Nature 459, 556–559 (2009).
Davidson, E. A. & Janssens, I. A. Temperature sensitivity of soil carbon decomposition and feedbacks to climate change. Nature 440, 165–173 (2006).
Karl, T. R. & Trenberth, K. E. Modern global climate change. Science 302, 1719–1723 (2003).
Huang, M. et al. Velocity of change in vegetation productivity over northern high latitudes. Nat. Ecol. Evol. 1, 1649–1654 (2017).
Fu, Y. H. et al. Declining global warming effects on the phenology of spring leaf unfolding. Nature 526, 104–107 (2015).
Piao, S. et al. Weakening temperature control on the interannual variations of spring carbon uptake across northern lands. Nat. Clim. Change 7, 359–363 (2017).
Keenan, T. F. & Riley, W. J. Greening of the land surface in the world’s cold regions consistent with recent warming. Nat. Clim. Change 8, 825–828 (2018).
Shen, M. et al. No evidence of continuously advanced green-up dates in the Tibetan Plateau over the last decade. Proc. Natl Acad. Sci. USA 110, E2329–E2329 (2013).
Zhang, G. et al. Mapping paddy rice planting areas through time series analysis of MODIS land surface temperature and vegetation index data. ISPRS J. Photogramm. Remote Sens. 106, 157–171 (2015).
Zhang, Y. et al. Consistency between sun-induced chlorophyll fluorescence and gross primary production of vegetation in North America. Remote Sens. Environ. 183, 154–169 (2016).
Frankenberg, C. et al. New global observations of the terrestrial carbon cycle from GOSAT: patterns of plant fluorescence with gross primary productivity. Geophys. Res. Lett. 38, 1–6 (2011).
Zhang, Y. et al. On the relationship between sub-daily instantaneous and daily total gross primary production: implications for interpreting satellite-based SIF retrievals. Remote Sens. Environ. 205, 276–289 (2018).
Joiner, J. et al. Global monitoring of terrestrial chlorophyll fluorescence from moderate-spectral-resolution near-infrared satellite measurements: methodology, simulations, and application to GOME-2. Atmos. Meas. Tech. 6, 2803–2823 (2013).
Köhler, P. et al. Global retrievals of solar-induced chlorophyll fluorescence with TROPOMI: first results and intersensor comparison to OCO-2. Geophys. Res. Lett. 45, 10456–10463 (2018).
Zhang, Y., Joiner, J., Gentine, P. & Zhou, S. Reduced solar-induced chlorophyll fluorescence from GOME-2 during Amazon drought caused by dataset artifacts. Glob. Change Biol. 24, 2229–2230 (2018).
Reichstein, M. et al. On the separation of net ecosystem exchange into assimilation and ecosystem respiration: review and improved algorithm. Glob. Change Biol. 11, 1424–1439 (2005).
Dušek, J., Čížková, H., Stellner, S., Czerný, R. & Květ, J. Fluctuating water table affects gross ecosystem production and gross radiation use efficiency in a sedge-grass marsh. Hydrobiologia 692, 57–66 (2012).
Pugh, C. A., Reed, D. E., Desai, A. R. & Sulman, B. N. Wetland flux controls: how does interacting water table levels and temperature influence carbon dioxide and methane fluxes in northern Wisconsin? Biogeochemistry 137, 15–25 (2018).
Migliavacca, M. et al. Using digital repeat photography and eddy covariance data to model grassland phenology and photosynthetic CO2 uptake. Agric. For. Meteorol. 151, 1325–1337 (2011).
Roerink, G. J., Menenti, M. & Verhoef, W. Reconstructing cloudfree NDVI composites using Fourier analysis of time series. Int. J. Remote Sens. 21, 1911–1917 (2000).
Piao, S., Fang, J., Zhou, L., Ciais, P. & Zhu, B. Variations in satellite-derived phenology in China’s temperate vegetation. Glob. Change Biol. 12, 672–685 (2006).
Gonsamo, A., Chen, J. M. & D’Odorico, P. Deriving land surface phenology indicators from CO2 eddy covariance measurements. Ecol. Indic. 29, 203–207 (2013).
Gu, L. Response of a deciduous forest to the Mount Pinatubo eruption: enhanced photosynthesis. Science 299, 2035–2038 (2003).
Sims, D. A. et al. Parallel adjustments in vegetation greenness and ecosystem CO2 exchange in response to drought in a Southern California chaparral ecosystem. Remote Sens. Environ. 103, 289–303 (2006).
Source: Ecology - nature.com