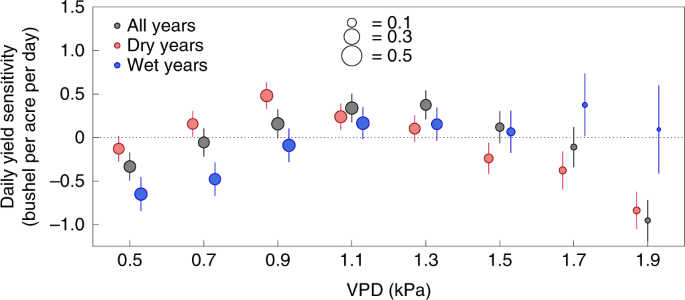
Butler, E. E. & Huybers, P. Variations in the sensitivity of US maize yield to extreme temperatures by region and growth phase. Environ. Res. Lett. 10, 034009 (2015).
Handmer, J. et al. in Managing the Risks of Extreme Events and Disasters to Advance Climate Change Adaptation (eds Field, C. B. et al.) 231–290 (IPCC, Cambridge Univ. Press, 2012).
Lobell, D. B., Bänziger, M., Magorokosho, C. & Vivek, B. Nonlinear heat effects on African maize as evidenced by historical yield trials. Nat. Clim. Change 1, 42–45 (2011).
Lobell, D. B. et al. The critical role of extreme heat for maize production in the United States. Nat. Clim. Change 3, 497–501 (2013).
Porter, J. R. et al. in Climate Change 2014: Impacts, Adaptation, and Vulnerability. (eds Field, C. B. et al.) 485–533 (IPCC, Cambridge Univ. Press, 2014).
Schlenker, W. & Roberts, M. J. Nonlinear temperature effects indicate severe damages to U.S. crop yields under climate change. Proc. Natl Acad. Sci. USA 106, 15594–15598 (2009).
Zhao, C. et al. Temperature increase reduces global yields of major crops in four independent estimates. Proc. Natl Acad. Sci. USA 114, 9326–9331 (2017).
Butler, E. E. & Huybers, P. Adaptation of US maize to temperature variations. Nat. Clim. Change 3, 68–72 (2013).
Basso, B. & Ritchie, J. T. Temperature and drought effects on maize yield. Nat. Clim. Change 4, 233 (2014).
Fezzi, C. & Bateman, I. The impact of climate change on agriculture: nonlinear effects and aggregation bias in Ricardian models of farmland values. J. Assoc. Environ. Resour. Econ. 2, 57–92 (2015).
Anderson, C. J., Babcock, B. A., Peng, Y., Gassman, P. W. & Campbell, T. D. Placing bounds on extreme temperature response of maize. Environ. Res. Lett. 10, 124001 (2015).
Carter, E. K., Melkonian, J., Riha, S. J. & Shaw, S. B. Separating heat stress from moisture stress: analyzing yield response to high temperature in irrigated maize. Environ. Res. Lett. 11, 094012 (2016).
Carter, E. K., Melkonian, J., Steinschneider, S. & Riha, S. J. Rainfed maize yield response to management and climate covariability at large spatial scales. Agric. For. Meteorol. 256–257, 242–252 (2018).
Rosenzweig, C., Tubiello, F. N., Goldberg, R., Mills, E. & Bloomfield, J. Increased crop damage in the US from excess precipitation under climate change. Global Environ. Change 12, 197–202 (2002).
Schauberger, B. et al. Consistent negative response of US crops to high temperatures in observations and crop models. Nat. Commun. 8, 1–9 (2017).
Shaw, S. B., Mehta, D. & Riha, S. J. Using simple data experiments to explore the influence of non-temperature controls on maize yields in the mid-West and Great Plains. Clim. Change 122, 747–755 (2014).
Troy, T. J., Kipgen, C. & Pal, I. The impact of climate extremes and irrigation on US crop yields. Environ. Res. Lett. 10, 054013 (2015).
Urban, D. W., Sheffield, J. & Lobell, D. B. The impacts of future climate and carbon dioxide changes on the average and variability of US maize yields under two emission scenarios. Environ. Res. Lett. 10, 045003 (2015).
Siebert, S., Webber, H., Zhao, G. & Ewert, F. Heat stress is overestimated in climate impact studies for irrigated agriculture. Environ. Res. Lett. 12, 054023 (2017).
Siebert, S., Ewert, F., Eyshi Rezaei, E., Kage, H. & Graß, R. Impact of heat stress on crop yield—on the importance of considering canopy temperature. Environ. Res. Lett. 9, 044012 (2014).
Webber, H. et al. Simulating canopy temperature for modelling heat stress in cereals. Environ. Model. Softw. 77, 143–155 (2016).
Kaur, G., Zurweller, B. A., Nelson, K. A., Motavalli, P. P. & Dudenhoeffer, C. J. Soil waterlogging and nitrogen fertilizer management effects on corn and soybean yields. Agron. J. 109, 97–106 (2017).
Ortiz-Bobea, A., Wang, H., Carrillo, C. M. & Ault, T. R. Unpacking the climatic drivers of US agricultural yields. Environ. Res. Lett. 14, 064003–064013 (2019).
Li, Y., Guan, K., Schnitkey, G. D., DeLucia, E. & Peng, B. Excessive rainfall leads to maize yield loss of a comparable magnitude to extreme drought in the United States. Global Change Biol. 5, 143–113 (2019).
Entekhabi, D. et al. The Soil Moisture Active Passive (SMAP) mission. Proc. IEEE 98, 704–716 (2010).
Hsiao, J., Swann, A. L. S. & Kim, S.-H. Maize yield under a changing climate: the hidden role of vapor pressure deficit. Agric. For. Meteorol. 279, 107692 (2019).
Seneviratne, S. I. et al. Investigating soil moisture–climate interactions in a changing climate: a review. Earth Sci. Rev. 99, 125–161 (2010).
Novick, K. A. et al. The increasing importance of atmospheric demand for ecosystem water and carbon fluxes. Nat. Clim. Change 6, 1023–1027 (2016).
Tao, F., Yokozawa, M. & Zhang, Z. Modelling the impacts of weather and climate variability on crop productivity over a large area: a new process-based model development, optimization, and uncertainties analysis. Agric. For. Meteorol. 149, 831–850 (2009).
Suyker, A. AmeriFlux US-Ne3 Mead – Rainfed Maize-Soybean Rotation Site from 2001–Present (AmeriFlux, 2016); http://ameriflux.lbl.gov/sites/siteinfo/US-Ne3
Kramer, P. J. & Boyer, J. S. Water Relations of Plants and Soils (Elsevier, 1995).
Bennett, J. M., Sinclair, T. R., Muchow, R. C. & Costello, S. R. Dependence of stomatal conductance on leaf water potential, turgor potential, and relative water content in field-grown soybean and maize. Crop Sci. 27, 984–990 (1987).
Cochard, H. Xylem embolism and drought-induced stomatal closure in maize. Planta 215, 466–471 (2002).
Farquhar, G. D. & Sharkey, T. D. Stomatal conductance and photosynthesis. Annu. Rev. Plant Physiol. 33, 317–345 (1982).
Wenkert, W., Fausey, N. R. & Watters, H. D. Flooding responses in Zea mays L. Plant Soil 62, 351–366 (1981).
Yordanova, R. Y. & Popova, L. P. Flooding-induced changes in photosynthesis and oxidative status in maize plants. Acta Physiol. Plant. 29, 535–541 (2007).
Voesenek, L. A. C. J. & Bailey-Serres, J. Flood adaptive traits and processes: an overview. New Phytol. 206, 57–73 (2015).
National Agricultural Statistics Service (US Department of Agriculture, 2019); https://www.nass.usda.gov
Cui, C. et al. Soil moisture mapping from satellites: an intercomparison of SMAP, SMOS, FY3B, AMSR2, and ESA CCI over two dense network regions at different spatial scales. Remote Sens. 10, 33–19 (2018).
Ma, H. et al. Satellite surface soil moisture from SMAP, SMOS, AMSR2 and ESA CCI: a comprehensive assessment using global ground-based observations. Remote Sens. Environ. 231, 111215 (2019).
Albergel, C. et al. From near-surface to root-zone soil moisture using an exponential filter: an assessment of the method based on in-situ observations and model simulations. Hydrol. Earth Syst. Sci. 12, 1323–1337 (2008).
Ficklin, D. L. & Novick, K. A. Historic and projected changes in vapor pressure deficit suggest a continental-scale drying of the United States atmosphere. J. Geophys. Res. Atmos. 122, 2061–2079 (2017).
Berg, A., Sheffield, J. & Milly, P. C. D. Divergent surface and total soil moisture projections under global warming. Geophys. Res. Lett. 44, 236–244 (2017).
Ukkola, A. M. et al. Evaluating CMIP5 model agreement for multiple drought metrics. J. Hydrometeorol. 19, 969–988 (2018).
Kell, D. B. Breeding crop plants with deep roots: their role in sustainable carbon, nutrient and water sequestration. Ann. Bot. 108, 407–418 (2011).
Messina, C. D. et al. Limited-transpiration trait may increase maize drought tolerance in the US corn belt. Agron. J. 107, 1978 (2015).
Daly, C. et al. Physiographically sensitive mapping of climatological temperature and precipitation across the conterminous United States. Int. J. Climatol. 28, 2031–2064 (2008).
Nicoullaud, B., King, D. & Tardieu, F. Vertical distribution of maize roots in relation to permanent soil characteristics. Plant Soil 159, 245–254 (1994).
Ford, T. W., Harris, E. & Quiring, S. M. Estimating root zone soil moisture using near-surface observations from SMOS. Hydrol. Earth Syst. Sci. 18, 139–154 (2014).
Bell, J. E. et al. U.S. climate reference network soil moisture and temperature observations. J. Hydrometeorol. 14, 977–988 (2013).
Diamond, H. J. et al. U.S. climate reference network after one decade of operations: status and assessment. Bull. Am. Meteorol. Soc. 94, 485–498 (2013).
Slaets, J. I. F., Piepho, H.-P., Schmitter, P., Hilger, T. & Cadisch, G. Quantifying uncertainty on sediment loads using bootstrap confidence intervals. Hydrol. Earth Syst. Sci. 21, 571–588 (2017).
Leakey, A. D. B. et al. Elevated CO2 effects on plant carbon, nitrogen, and water relations: six important lessons from FACE. J. Exp. Bot. 60, 2859–2876 (2009).
Source: Resources - nature.com