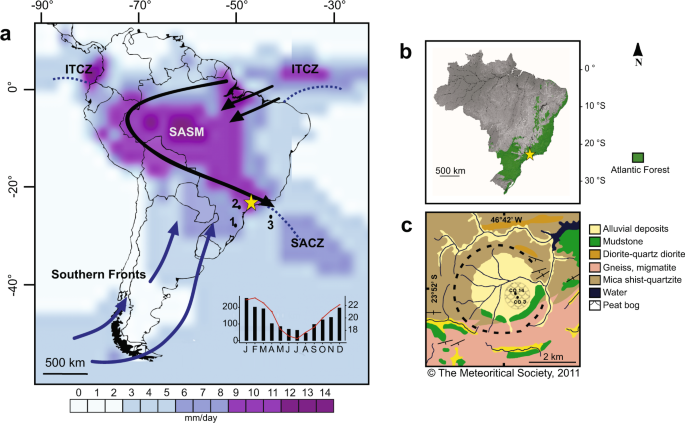
Lewis, S. L. Tropical forests and the changing earth system. Philos. Trans. R. Soc. B Biol. Sci. 361, 195–210 (2006).
Saatchi, S. S. et al. Benchmark map of forest carbon stocks in tropical regions across three continents. Proc. Natl. Acad. Sci. 108, 9899–9904 (2011).
Malhi, Y., Meir, P. & Brown, S. Forests, carbon and global climate. Philos. Trans. R. Soc. Lond. Ser. Math. Phys. Eng. Sci. 360, 1567–1591 (2002).
Marengo, J. A. On the hydrological cycle of the Amazon basin: a historical review and current State-of-the-art. Rev. bras. meteorol. 21, 1–19 (2006).
Levine, N. M. et al. Ecosystem heterogeneity determines the ecological resilience of the Amazon to climate change. Proc. Natl. Acad. Sci. 113, 793–797 (2016).
Duarte, L. D. S., Bergamin, R. S., Marcilio-Silva, V., Seger, G. D. D. S. & Marques, M. C. M. Phylobetadiversity among forest types in the Brazilian Atlantic forest complex. PLoS ONE 9, e105043 (2014).
Cheng, H. et al. Climate change patterns in Amazonia and biodiversity. Nat. Commun. 4, 1411 (2013).
Stríkis, N. M. et al. South American monsoon response to iceberg discharge in the North Atlantic. Proc. Natl. Acad. Sci. 115, 3788–3793 (2018).
Cruz, F. W. et al. Insolation-driven changes in atmospheric circulation over the past 116,000 years in subtropical Brazil. Nature 434, 63–66 (2005).
Deininger, M., Ward, B. M., Novello, V. F. & Cruz, F. W. Late Quaternary variations in the South American monsoon system as inferred by speleothems—New perspectives using the SISAL database. Quaternary 2, 6 (2019).
Bennett, K., Bhagwat, S. & Willis, K. Neotropical refugia. The Holocene 22, 1207–1214 (2012).
Baker, P. A. & Fritz, S. C. Nature and causes of Quaternary climate variation of tropical South America. Quat. Sci. Rev. 124, 31–47 (2015).
Flantua, S. G. A. et al. Updated site compilation of the Latin American Pollen Database. Rev. Palaeobot. Palynol. 223, 104–115 (2015).
Kershaw, A. P., Bretherton, S. C. & van der Kaars, S. A complete pollen record of the last 230 ka from Lynch’s Crater, north-eastern Australia. Palaeogeogr. Palaeoclimatol. Palaeoecol. 251, 23–45 (2007).
Miller, C. S., Gosling, W. D., Kemp, D. B., Coe, A. L. & Gilmour, I. Drivers of ecosystem and climate change in tropical West Africa over the past ∼540,000 years. J. Quat. Sci. 31, 671–677 (2016).
D’Apolito, C., Absy, M. L. & Latrubesse, E. M. The movement of pre-adapted cool taxa in north-central Amazonia during the last glacial. Quat. Sci. Rev. 169, 1–12 (2017).
Ledru, M.-P., Mourguiart, P. & Riccomini, C. Related changes in biodiversity, insolation and climate in the Atlantic rainforest since the last interglacial. Palaeogeogr. Palaeoclimatol. Palaeoecol. 271, 140–152 (2009).
Litt, T., Pickarski, N., Heumann, G., Stockhecke, M. & Tzedakis, P. C. A 600,000 year long continental pollen record from Lake Van, eastern Anatolia (Turkey). Quat. Sci. Rev. 104, 30–41 (2014).
Bard, E. & Rickaby, R. E. M. Migration of the subtropical front as a modulator of glacial climate. Nature 460, 380–383 (2009).
Khodri, M., Kageyama, M. & Roche, D. M. Sensitivity of South American tropical climate to Last Glacial Maximum boundary conditions: Focus on teleconnections with tropics and extratropics In Past Climate Variability in South America and Surrounding Regions (eds. Vimeux, F., Sylvestre, F. & Khodri, M.).14, 213–238 (Springer Netherlands, 2009).
Vimeux, F., Masson, V., Jouzel, J., Stievenard, M. & Petit, J. R. Glacial–interglacial changes in ocean surface conditions in the Southern Hemisphere. Nature 398, 410–413 (1999).
Masson-Delmotte, V. et al. EPICA dome C record of glacial and interglacial intensities. Quat. Sci. Rev. 29, 113–128 (2010).
Fogwill, C. J., Turney, C. S. M., Hutchinson, D. K., Taschetto, A. S. & England, M. H. Obliquity control on southern hemisphere climate during the last glacial. Sci. Rep. 5, 11673 (2015).
Riccomini, C. et al. The Colônia structure, São Paulo, Brazil. Meteorit. Planet. Sci. 46, 1630–1639 (2011).
Ledru, M.-P. et al. Why deep drilling in the Colônia Basin (Brazil)? Sci. Drill. 20, 33–39 (2015).
Grimm, A., Ferraz, S. & Gomes, J. Precipitation anomalies in Southern Brazil associated with El Niño and La Niña events. Am. Meteorol. Soc. 11, 2863–2880 (1998).
Garreaud, R. D. Cold air incursions over subtropical South America: Mean structure and dynamics. Mon Weather Rev. 128, 2544–2559 (2000).
Garreaud, R. D., Vuille, M., Compagnucci, R. & Marengo, J. Present-day South American climate. Palaeogeogr. Palaeoclimatol. Palaeoecol. 281, 180–195 (2009).
DAEE. Portal do departamento de águas e energia elétrica. http://www.hidrologia.daee.sp.gov.br/ (2019)
INMET. Instituto nacional de meteorologia. http://www.inmet.gov.br (2019)
Locarnini, R. A. et al. World ocean atlas 2018. (ed. Mishonov, A.) 1, 52 (NOAA Atlas NESDIS, 2019).
Garcia, R. J. F. & Pirani, J. R. Análise florística, ecológica e fitogeográfica do núcleo Curucutu, parque estadual da Serra do Mar (São Paulo, SP), com ênfase nos campos junto à crista da Serra do Mar. Hoehnea 32, 1–48 (2005).
Ledru, M.-P., Montade, V., Blanchard, G. & Hély, C. Long-term spatial changes in the distribution of the Brazilian Atlantic forest. Biotropica 48, 159–169 (2016).
Montade, V. et al. A new modern pollen dataset describing the Brazilian Atlantic forest. The Holocene 29, 1253–1262 (2019).
Naafs, B. D. A. et al. Introducing global peat-specific temperature and pH calibrations based on brGDGT bacterial lipids. Geochim. Cosmochim. Acta 208, 285–301 (2017).
Blaauw, M. & Christen, J. A. Flexible paleoclimate age-depth models using an autoregressive gamma process. Bayesian Anal. 6, 457–474 (2011).
Laj, C., Guillou, H. & Kissel, C. Dynamics of the earth magnetic field in the 10–75 kyr period comprising the Laschamp and Mono Lake excursions: New results from the French Chaîne des Puys in a global perspective. Earth Planet. Sci. Lett. 387, 184–197 (2014).
Lisiecki, L. E. & Raymo, M. E. A Pliocene-Pleistocene stack of 57 globally distributed benthic δ 18 O records. Paleoceanography 20, 1–17 (2005).
Santos, T. P. et al. Prolonged warming of the Brazil Current precedes deglaciations. Earth Planet. Sci. Lett. 463, 1–12 (2017).
Kohfeld, K. E. et al. Southern hemisphere westerly wind changes during the Last Glacial Maximum: paleo-data synthesis. Quat. Sci. Rev. 68, 76–95 (2013).
Cárdenas, M. L., Wilson, O. J., Schorn, L. A., Mayle, F. E. & Iriarte, J. A quantitative study of modern pollen–vegetation relationships in southern Brazil’s Araucaria forest. Rev. Palaeobot. Palynol. 265, 27–40 (2019).
Cruz, F. W., Burns, S. J., Karmann, I., Sharp, W. D. & Vuille, M. Reconstruction of regional atmospheric circulation features during the late Pleistocene in subtropical Brazil from oxygen isotope composition of speleothems. Earth Planet. Sci. Lett. 248, 495–507 (2006).
Burns, S. J., Welsh, L. K., Scroxton, N., Cheng, H. & Edwards, R. L. Millennial and orbital scale variability of the South American monsoon during the penultimate glacial period. Sci. Rep. 9, 1234 (2019).
Toggweiler, J. R., Russell, J. L. & Carson, S. R. Midlatitude westerlies, atmospheric CO2, and climate change during the ice ages: westerlies and CO2 during the ice ages. Paleoceanography 21, 1–15 (2006).
de Boer, B., Lourens, L. J. & van de Wal, R. S. W. Persistent 400,000-year variability of Antarctic ice volume and the carbon cycle is revealed throughout the Plio-Pleistocene. Nat. Commun. 5, 2999 (2014).
Gu, F. et al. Long-term vegetation, climate and ocean dynamics inferred from a 73,500 years old marine sediment core (GeoB2107-3) off southern Brazil. Quat. Sci. Rev. 172, 55–71 (2017).
Bosmans, J. H. C., Hilgen, F. J., Tuenter, E. & Lourens, L. J. Obliquity forcing of low-latitude climate. Clim. Past 11, 1335–1346 (2015).
Yin, Q. & Berger, A. Interglacial analogues of the Holocene and its natural near future. Quat. Sci. Rev. 120, 28–46 (2015).
Denton, G. H. et al. The last glacial termination. Science 328, 1652–1656 (2010).
Menviel, L. et al. Southern hemisphere westerlies as a driver of the early deglacial atmospheric CO2 rise. Nat. Commun. 9, 2503 (2018).
Hirata, F. E. & Grimm, A. M. The role of synoptic and intraseasonal anomalies on the life cycle of rainfall extremes over South America: non-summer conditions. Clim. Dyn. 49, 313–326 (2017).
Marengo, J., Cornejo, A., Satyamurty, P. & Nobre, C. Cold surges in tropical and extratropical South America: The strong event in June 1994. Mon. Wea. Rev 125, 2759–2786 (1997).
Grimm, A. M. Interannual climate variability in South America: impacts on seasonal precipitation, extreme events, and possible effects of climate change. Stoch. Environ. Res. Risk Assess. 25, 537–554 (2011).
Reimer, P. J. et al. IntCal13 and Marine13 radiocarbon age calibration curves 0–50,000 years cal BP. Radiocarbon 55, 1869–1887 (2013).
Hua, Q., Barbetti, M. & Rakowski, A. Z. Atmospheric radiocarbon for the period 1950–2010. Radiocarbon 55, 2059–2072 (2013).
R Development Core Team. R: A Language and Environment for Statistical Computing. (R Foundation for Statistical Computing, Vienna, Austria, 2019).
Aitken, M. J. An introduction to optical dating: the dating of Quaternary sediments by the use of photon-stimulated luminescence. (Oxford University Press, 1998).
Murray, A. S. & Wintle, A. G. The single aliquot regenerative dose protocol: potential for improvements in reliability. Radiat. Meas. 37, 377–381 (2003).
Jain, M., Duller, G. A. T. & Wintle, A. G. Dose response, thermal stability and optical bleaching of the 310 °C isothermal TL signal in quartz. Radiat. Meas. 42, 1285–1293 (2007).
Buylaert, J.-P. et al. A robust feldspar luminescence dating method for middle and late Pleistocene sediments: Feldspar luminescence dating of middle and late Pleistocene sediments. Boreas 41, 435–451 (2012).
Galbraith, R. F., Roberts, R. G., Laslett, G. M., Yoshida, H. & Olley, J. M. Optical dating of single and multiple grains of quartz from minmium rock shelter, northern Australia: part i, experimental design and statistical models. Archaeometry 41, 339–364 (1999).
Guérin, G., Mercier, N. & Adamiec, G. Dose-rate conversion factors: update. Ancient TL 29, 5–8 (2011).
Prescott, J. & Stephan, L. The contribution of cosmic radiation to the environmental dose for thermoluminescent dating, latitude, altitude and depth dependences. PACT 6, 17–25 (1982).
Channell, J. E. T., Xuan, C. & Hodell, D. A. Stacking paleointensity and oxygen isotope data for the last 1.5 Myr (PISO-1500). Earth Planet. Sci. Lett. 283, 14–23 (2009).
Simon, Q. et al. Authigenic 10 Be/9 Be ratio signatures of the cosmogenic nuclide production linked to geomagnetic dipole moment variation since the Brunhes/Matuyama boundary. J. Geophys. Res. Solid Earth 121, 7716–7741 (2016).
Simon, Q., St‐Onge, G. & Hillaire‐Marcel, C. Late Quaternary chronostratigraphic framework of deep Baffin Bay glaciomarine sediments from high‐resolution paleomagnetic data. Geochem. Geophys. Geosystems 13, 1–24 (2012).
Thouveny, N., Creer, K. M. & Blunk, I. Extension of the Lac du Bouchet palaeomagnetic record over the last 120,000 years. Earth Planet. Sci. Lett. 97, 140–161 (1990).
Croudace, I. W., Rindby, A. & Rothwell, R. G. ITRAX: description and evaluation of a new multi-function X-ray core scanner. Geol. Soc. Lond. Spec. Publ. 267, 51–63 (2006).
Chawchai, S., Kylander, M. E., Chabangborn, A., Löwemark, L. & Wohlfarth, B. Testing commonly used X-ray fluorescence core scanning-based proxies for organic-rich lake sediments and peat. Boreas 45, 180–189 (2016).
Hopmans, E. C., Schouten, S. & Sinninghe Damsté, J. S. The effect of improved chromatography on GDGT-based palaeoproxies. Org. Geochem. 93, 1–6 (2016).
Davtian, N., Bard, E., Ménot, G. & Fagault, Y. The importance of mass accuracy in selected ion monitoring analysis of branched and isoprenoid tetraethers. Org. Geochem. 118, 58–62 (2018).
Davtian, N., Ménot, G., Fagault, Y. & Bard, E. Western mediterranean sea paleothermometry over the last glacial cycle based on the novel RI-OH index. Paleoceanogr. Paleoclimatology 34, 616–634 (2019).
Sanchi, L., Ménot, G. & Bard, E. An automated purification method for archaeal and bacterial tetraethers in soils and sediments. Org. Geochem. 54, 83–90 (2013).
De Jonge, C. et al. Occurrence and abundance of 6-methyl branched glycerol dialkyl glycerol tetraethers in soils: Implications for palaeoclimate reconstruction. Geochim. Cosmochim. Acta 141, 97–112 (2014).
Russell, J. M., Hopmans, E. C., Loomis, S. E., Liang, J. & Sinninghe Damsté, J. S. Distributions of 5- and 6-methyl branched glycerol dialkyl glycerol tetraethers (brGDGTs) in East African lake sediment: Effects of temperature, pH, and new lacustrine paleotemperature calibrations. Org. Geochem. 117, 56–69 (2018).
Hopmans, E. C. et al. A novel proxy for terrestrial organic matter in sediments based on branched and isoprenoid tetraether lipids. Earth Planet. Sci. Lett. 224, 107–116 (2004).
Weijers, J. W. H., Schouten, S., Spaargaren, O. C. & Sinninghe Damsté, J. S. Occurrence and distribution of tetraether membrane lipids in soils: Implications for the use of the TEX86 proxy and the BIT index. Org. Geochem. 37, 1680–1693 (2006).
De Jonge, C. et al. Identification of novel penta- and hexamethylated branched glycerol dialkyl glycerol tetraethers in peat using HPLC–MS2, GC–MS and GC–SMB-MS. Org. Geochem. 54, 78–82 (2013).
Xiao, W. et al. Ubiquitous production of branched glycerol dialkyl glycerol tetraethers(brGDGTs) in global marine environments: a new source indicator for brGDGTs. Biogeosciences 13, 5883–5894 (2016).
Martin, C. et al. Early Holocene Thermal Maximum recorded by branched tetraethers and pollen in Western Europe (Massif Central, France). Quat. Sci. Rev. 228, 106109 (2020).
Stute, M. et al. Cooling of tropical Brazil (5 °C) during the Last Glacial Maximum. Science 269, 379–383 (1995).
Faegri, K. & Iversen, J. Textbook of Pollen Analysis (John Wiley & Sons, Chichester, 1989).
Bennett, K. Psimpoll 4.27: C program for plotting pollen diagrams and analysing pollen data. (Department of Archaeology and Palaeoecology, Queen’s University of Belfast, 2009).
Daniau, A.-L. et al. Orbital-scale climate forcing of grassland burning in southern Africa. Proc. Natl. Acad. Sci. 110, 5069–5073 (2013).
Adler, R. F. et al. The version-2 global precipitation climatology project (GPCP) monthly precipitation analysis (1979–Present). J. Hydrometeorol. 4, 1147–1167 (2003).
Laskar, J. et al. A long-term numerical solution for the insolation quantities of the Earth. Astron. Astrophys. 428, 261–285 (2004).
Railsback, L. B., Gibbard, P. L., Head, M. J., Voarintsoa, N. R. G. & Toucanne, S. An optimized scheme of lettered marine isotope substages for the last 1.0 million years, and the climatostratigraphic nature of isotope stages and substages. Quat. Sci. Rev. 111, 94–106 (2015).
Henderson, G. M. & Slowey, N. C. Evidence from U–Th dating against Northern Hemisphere forcing of the penultimate deglaciation. Nature 404, 61–66 (2000).
Lüthi, D. et al. High-resolution carbon dioxide concentration record 650,000–800,000 years before present. Nature 453, 379–382 (2008).
Source: Ecology - nature.com