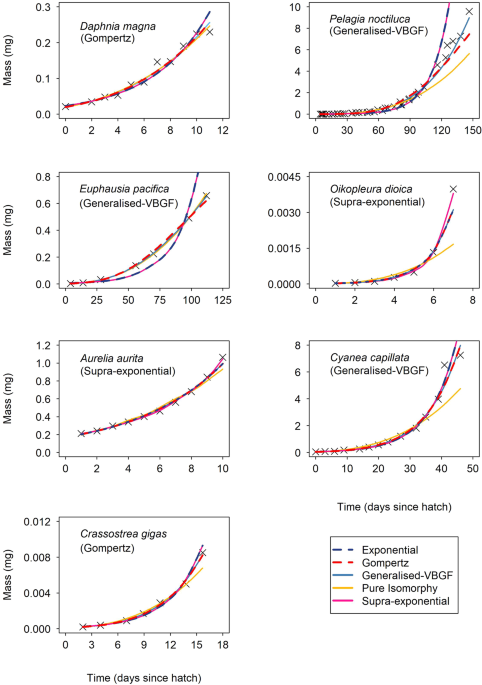
Holm, S. et al. A comparative perspective on longevity: the effect of body size dominates over ecology in moths. J. Evol. Biol. 29(12), 2422–2435 (2006).
Woodward, G. et al. Body size in ecological networks. Trends Ecol. Evol. 20(7), 402–409 (2005).
Kwapich, C.L. Valentini, G. & Hölldobler, B. The non-additive effects of body size on nest architecture in a polymorphic ant. Philos. Trans. R. Soc. Lon., B, Biol Sci, 373(1753), 20170235 (2018).
Mayer, M., Shine, R. & Brown, G. P. Bigger babies are bolder: effects of body size on personality of hatchling snakes. Behaviour 153(3), 313–323 (2016).
Mirth, C. K., Frankino, W. A. & Shingleton, A. W. Allometry and size control: what can studies of body size regulation teach us about the evolution of morphological scaling relationships? Curr. Opin. Insect. 13, 93–98 (2016).
Gutowsky et al. Interactive effects of sex and body size on the movement ecology of adfluvial bull trout (Salvelinus confluentus). Can. J. Zool. 94(1), 31–40 (2015).
Green, D. M. Implications of female body-size variation for the reproductive ecology of an anuran amphibian. Ethol. Ecol. Evol. 27(2), 173–184 (2015).
Davies, P. S. Physiological ecology of Patella. I. The effect of body size and temperature on metabolic rate. J. Mar. Biol. Assoc. UK 46(3), 647–658 (1966).
Illius, A. W. & Gordon, I. J. Modelling the nutritional ecology of ungulate herbivores: evolution of body size and competitive interactions. Oecologia 89(3), 428–434 (1992).
González-Wangüemert, M., Valente, S. & Aydin, M. Effects of fishery protection on biometry and genetic structure of two target sea cucumber species from the Mediterranean Sea. Hydrobiologia 743(1), 65–74 (2015).
Jackson, C. J. & Wang, Y. G. Modelling growth rate of Penaeus monodon Fabricius in intensively managed ponds: effects of temperature, pond age and stocking density. Aquac. Res 29(1), 27–36 (1998).
Ansah, Y. B. & Frimpong, E. A. Using model-based inference to select a predictive growth curve for farmed tilapia. N. Am. J. Aquac. 77(3), 281–288 (2015).
Sulardiono, B., Prayitno, S. B. & Hendrarto, I. B. The growth analysis of Stichopus vastus (Echinodermata: Stichopodidae) in Karimunjawa waters. J. Coast. Dev 15, 315–323 (2012).
Petersen, J. K. et al. Mussels as a tool for mitigation of nutrients in the marine environment. Mar. Pollut. Bull. 82(1–2), 137–143 (2014).
Bridges, T. C., Turner, L. W., Smith, E. M., Stahly, T. S. & Loewer, O. J. A mathematical procedure for estimating animal growth and body composition. Trans. ASAE 29(5), 1342–1347 (1986).
Kirkwood, G. P. Estimation of von Bertalanffy growth curve parameters using both length increment and age–length data. Can. J. Fish. Aquat. Sci. 40(9), 1405–1411 (1983).
Panik, M. J. Growth Curve Modelling: Theory and Applications (John Wiley & Sons, 2014).
Potthoff, R. F. & Roy, S. N. A generalized multivariate analysis of variance model usefulespecially for growth curve problems. Biometrika, 51(3-4), 313-326 (1964).
Richards, F. J. A flexible growth function for empirical use. J. Exp. Bot. 10(2), 290–301 (1959).
Strenio, J. F., Weisberg, H. I. & Bryk, A. S. Empirical Bayes estimation of individual growth curve parameters and their relationship to covariates. Biometrics 39(1), 71–86 (1983).
Higgins, R. M., Diogo, H. & Isidro, E. J. Modelling growth in fish with complex life histories. Rev. Fish Biol. Fish. 25(3), 449–462 (2015).
Chang, Y. J., Sun, C. L., Chen, Y. & Yeh, S. Z. Modelling the growth of crustacean species. Rev. Fish Biol. Fish. 22(1), 157–187 (2012).
Fuentes-Santos, I., Labarta, U., Arranz, K. & Fernández-Reiriz, M. J. From classical to nonparametric growth models: Towards comprehensive modelling of mussel growth patterns. Mar. Environ. Res. 127, 41–48 (2017).
Huchard, E. et al. Additive genetic variance and developmental plasticity in growth trajectories in a wild cooperative mammal. J. Evol. Biol. 27(9), 1893–1904 (2014).
Jager, T. & Ravagnan, E. Modelling growth of northern krill (Meganyctiphanes norvegica) using an energy-budget approach. Ecol. Model. 325, 28–34 (2016).
Marshall, D. J. & White, C. R. Have we outgrown the existing models of growth? Trends Ecol. Evol. 34(2), 102–111 (2018).
Quince, C. Abrams, P.A. Shuter, B.J. & Lester, N.P. Biphasic growth in fish I: theoretical foundations. J. Theor. Biol., 254(2), 197–206 (2008).
Derocher, A. E. & Wiig, Ø. Postnatal growth in body length and mass of polar bears (Ursus maritimus) at Svalbard. J. Zool. (Lond.) 256(3), 343–349 (2002).
Tjørve, K. M. C. & Tjørve, E. Shapes and functions of bird-growth models: how to characterise chick postnatal growth. Zoology 113(6), 326–333 (2010).
Ernsting, G., Zonneveld, C., Isaaks, J. A. & Kroon, A. Size at maturity and patterns of growth and reproduction in an insect with indeterminate growth. Oikos 66, 17–26 (1993).
Siegel, V. Age and growth of Antarctic Euphausiacea (Crustacea) under natural conditions. Mar. Biol. 96(4), 483–495 (1987).
Lehman, T. M. & Woodward, H. N. Modeling growth rates for sauropod dinosaurs. Paleobiology 34(2), 264–281 (2008).
Pütter, A. Studies on the physiological similarity. VI. Similarities in growth. Eur. J. Physiol. 180, 280 (1920).
Bertalanffy, Lvon Problems of organic growth. Nature 163(4135), 156–158 (1949).
Bertalanffy, Lvon A quantitative theory of organic growth (inquiries on growth laws. II). Hum. Biol. 10(2), 181–213 (1938).
Schnute, J. A versatile growth model with statistically stable parameters. Can. J. Fish. Aquat. Sci. 38(9), 1128–1140 (1981).
Góngora-Gómez, A. M., Leal-Sepúlveda, A. L., García-Ulloa, M., Aragón-Noriega, E. A. & Valenzuela-Quiñónez, W. Morphometric relationships and growth models for the oyster Crassostrea corteziensis cultivated at the southeastern coast of the Gulf of California Mexico. Lat. Am. J. Aquat. 46(4), 735–743 (2018).
Reynaga-Franco, F. J. et al. Multi-model inference as criterion to determine differences in growth patterns of distinct Crassostrea gigas stocks. Aquacul. Int. 27, 1–16 (2019).
Castillo-Vargasmachuca, S. G. Ponce-Palafox, J.T. Arámbul-Muñoz, E. Rodríguez-Domínguez, G. & Aragón-Noriega, E.A. The spotted rose snapper (Lutjanus guttatus Steindachner 1869) farmed in marine cages: review of growth models. Rev. Aquacult., 10(2), (2018).
Lugert, V., Tetens, J., Thaller, G., Schulz, C. & Krieter, J. Finding suitable growth models for turbot (Scophthalmus maximus L.) in aquaculture 1 (length application). Aquac. Res. 48(1), 24–36 (2017).
Yuancai, L., Marques, C. P. & Macedo, F. W. Comparison of Schnute’s and Bertalanffy-Richards’ growth functions. Forest Ecol. Manag. 96(3), 283–288 (1997).
Gompertz, B. On the nature of the function expressive of the law of human mortality, and on a new mode of determining the value of life contingencies. Phil. Trans. R. Soc. Lon. 115, 513–583 (1825).
Tjørve, K.M. & Tjørve, E. The use of Gompertz models in growth analyses, and new Gompertz-model approach: An addition to the Unified-Richards family. PLoS ONE, 12(6), (2017).
Alldredge, A. L. & Madin, L. P. Pelagic tunicates: unique herbivores in the marine plankton. Bioscience 32(8), 655–663 (1982).
West, G. B., Brown, J. H. & Enquist, B. J. A general model for the origin of allometric scaling laws in biology. Science 276(5309), 122–126 (1997).
Barneche, D. R. & Allen, A. P. The energetics of fish growth and how it constrains food-web trophic structure. Ecol. Lett., 21(6) (2018).
West, G. B., Brown, J. H. & Enquist, B. J. A general model for ontogenetic growth. Nature 413(6856), 628–631 (2001).
Moses, M. E. et al. Revisiting a model of ontogenetic growth: estimating model parameters from theory and data. Am. Nat. 171(5), 632–645 (2008).
HirstA.G. & Forster, J. When growth models are not universal: evidence from marine invertebrates. Proc. Biol. Sci., 280(1768), (2013).
Verhulst, P. F. Notice sur la loi que la population suit dans son accroissement. Corresp. Mathématique Phys 10, 113–21 (1839).
Katsanevakis, S. Modelling fish growth: model selection, multi-model inference and model selection uncertainty. Fish. Res. 81(2-3), 229–235 (2006).
Shi, P. J. et al. On the 3/4-exponent von Bertalanffy equation for ontogenetic growth. Ecol. Model. 276, 23–28 (2014).
Schnute, J. & Fournier, D. A new approach to length–frequency analysis: growth structure. Can. J. Fish. Aquat. Sci. 37(9), 1337–1351 (1980).
Kvålseth, T. O. Cautionary note about R-squared. Am. Stat. 39(4), 279–285 (1985).
Willett, J. B. & Singer, J. D. Another cautionary note about R-squared: Its use in weighted least-squares regression analysis. Am. Stat., 42(3), 236-238 (1988).
Maino, J. L. & Kearney, M. R. Ontogenetic and interspecific scaling of consumption in insects. Oikos, 124(12), 695-701 (2015).
Maino, J. L. & Kearney, M. R. Testing mechanistic models of growth in insects. Proc. Soc. Biol. Sci., 282(1819), 20151973 (2015).
Rosenfeld, J., Van Leeuwen, T., Richards, J. & Allen, D. Relationship between growth and standard metabolic rate: measurement artefacts and implications for habitat use and life‐history adaptation in salmonids. J. Anim. Ecol. 84(1), 4–20 (2015).
Killen, S. S., Atkinson, D. & Glazier, D. S. The intraspecific scaling of metabolic rate with body mass in fishes depends on lifestyle and temperature. Ecol. Lett 13(2), 184–193 (2010).
Ellenby, C. Body size in relation to oxygen consumption and pleopod beat in Ligia oceanica L. J. Exp. Biol. 28(4), 492–507 (1951).
Glazier, D.S. Hirst, A.G. & Atkinson, D. Shape shifting predicts ontogenetic changes inmetabolic scaling in diverse aquatic invertebrates. Proc. Biol. Sci., 282(1802), (2015).
Hirst, A. G., Glazier, D. S. & Atkinson, D. Body shape-shifting during growth permits tests that distinguish between competing geometric theories of metabolic scaling. Ecol. Lett. 17(10), 1274–1281 (2014).
Hirst, A. G. Intraspecific scaling of mass to length in pelagic animals: Ontogenetic shape change and its implications. Limnol. Oceanogr. 57(5), 1579–1590 (2012).
Kooijman, S. A. L. M. Dynamic Energy Budgets in Biological Systems (Cambridge University Press, 1993).
Kooijman, S. A. L. M. Dynamic Energy and Mass Budgets in Biological Systems (Cambridge University Press, 2000).
Ohnishi, S., Yamakawa, T. & Akamine, T. On the analytical solution for the Pütter – Bertalanffy growth equation. J. Theor. Biol. 343, 174–177 (2014).
Charnov, E. L. Fish growth: Bertalanffy k is proportional to reproductive effort. Environ. Biol. Fish. 83(2), 185–187 (2008).
Lester, N. P., Shuter, B. J. & Abrams, P. A. Interpreting the von Bertalanffy model of somatic growth in fishes: the cost of reproduction. Proc. Soc. Biol. Sci. 271(1548), 1625–1631 (2004).
Armstrong, D. P., Keevil, M. G., Rollinson, N. & Brooks, R. J. Subtle individual variation in indeterminate growth leads to major variation in survival and lifetime reproductive output in a long lived reptile. Funct. Ecol. 32(3), 752–761 (2017).
Moore, D. W. & Farrar, J. D. Effect of growth on reproduction in the freshwater amphipod, Hyalella azteca (Saussure). Hydrobiologia 328(2), 127–134 (1996).
Bouchard, L. & Winkler, G. Life cycle, growth and reproduction of Neomysis americana in the St. Lawrence estuarine transition zone. J. Plankton Res 40(6), 693–707 (2018).
Quesnel, L., King, W. J., Coulson, G. & Festa-Bianchet, M. Tall young females get ahead: size-specific fecundity in wild kangaroos suggests a steep trade-off with growth. Oecologia 186(1), 59–71 (2018).
Rollo, C. D. Growth negatively impacts the life span of mammals. Evol. Dev. 4(1), 55–61 (2002).
Bruce, R. C. Relative growth rates in three species of Desmognathus (Amphibia: Plethodontidae). Herpetologica 72(3), 174–180 (2016).
Pardo, S. A., Cooper, A. B. & Dulvy, N. K. Avoiding fishy growth curves. Methods Ecol. Evol. 4(4), 353–360 (2013).
Glazier, D. S. The 3/4-power law is not universal: evolution of isometric, ontogenetic metabolic scaling in pelagic animals. BioScience 56(4), 325–332 (2006).
Bhowmick, A. R., Chattopadhyay, G. & Bhattacharya, S. Simultaneous identification of growth law and estimation of its rate parameter for biological growth data: a new approach. J. Biol. Phys. 40(1), 71–95 (2014).
L’Abée-Lund, J. H., Langeland, A., Jonsson, B. & Ugedal, O. Spatial segregation by age and size in Arctic charr: a trade-off between feeding possibility and risk of predation. J. Anim. Ecol. 62, 160–168 (1993).
Tan, H., Hirst, A. G., Glazier, D. S. & Atkinson, D. Ecological pressures and the contrasting scaling of metabolism and body shape in coexisting taxa: cephalopods versus teleost fish. Philos. Trans. R. Soc. Lon., B, Biol. Sci. 374(1778), 20180543 (2019).
Seibel, B. A., Thuesen, E. V., Childress, J. J. & Gorodezky, L. A. Decline in pelagic cephalopod metabolism with habitat depth reflects differences in locomotory efficiency. Biol. Bull. 192(2), 262–278 (1997).
Mitchell, S. F., Trainor, F. R., Rich, P. H. & Goulden, C. E. Growth of Daphnia magna in the laboratory in relation to the nutritional state of its food species, Chlamydomonas reinhardtii. J. Plankton Res. 14(3), 379–391 (1992).
Lilley, M. K. et al. Culture and growth of the jellyfish Pelagia noctiluca in the laboratory. Mar. Ecol. Prog. Ser. 510, 265–273 (2014).
Ross, R. M. Energetics of Euphausia pacifica. II. Complete carbon and nitrogen budgets at 8 and 12 °C throughout the life span. Mar. Biol. 68(1), 15–23 (1982).
Lombard, F., Renaud, F., Sainsbury, C., Sciandra, A. & Gorsky, G. Appendicularian ecophysiology I: Food concentration dependent clearance rate, assimilation efficiency, growth and reproduction of Oikopleura dioica. J. Mar. Sys 78(4), 606–616 (2009).
Båmstedt, U., Wild, B. & Martinussen, M. Significance of food type for growth of ephyrae Aurelia aurita (Scyphozoa). Mar. Biol. 139(4), 641–650 (2001).
Båmstedt, U., Ishii, H. & Martlnussen, M. B. Is the scyphomedusa Cyanea capillata (l.) dependent on gelatinous prey for its early development? Sarsia 82(3), 269–273 (1997).
Kheder, R. B., Quéré, C., Moal, J. & Robert, R. Effect of nutrition on Crassostrea gigas larval development and the evolution of physiological indices. Part A: Quantitative and qualitative diet effects. Aquaculture 305(1–4), 165–173 (2010).
Thomsen, J., Casties, I., Pansch, C., Körtzinger, A. & Melzner, F. Food availability outweighs ocean acidification effects in juvenile Mytilus edulis: laboratory and field experiments. Glob. Chang. Biol. 19(4), 1017–1027 (2013).
Domingues, P. M., Sykes, A. & Andrade, J. P. The effects of temperature in the life cycle of two consecutive generations of the cuttlefish Sepia officinalis (Linnaeus, 1758), cultured in the Algarve (South Portugal). Aquacult. Int 10(3), 207–220 (2002).
Maranhão, P. & Marques, J. C. The influence of temperature and salinity on the duration of embryonic development, fecundity and growth of the amphipod Echinogammarus marinus Leach (Gammaridae). Acta Oecol. 24(1), 5–13 (2003).
Stumpf, L., Tropea, C. & Greco, L. S. L. Recovery growth of Cherax quadricarinatus juveniles fed on two high-protein diets: Effect of daily feeding following a cyclic feeding period on growth, biochemical composition and activity of digestive enzymes. Aquaculture 433, 404–410 (2014).
Ito, M. & Lucas, J. S. The Complete Larval Development of the Scyllarid Lobster, Scyllarus demani holthuis, 1946 (Decapoda, Scyllaridae), in the Laboratory. Crustaceana 58(2), 144–167 (1990).
Ibánez, C. M. & Keyl, F. Cannibalism in cephalopods. Rev. Fish Biol. Fish. 20(1), 123–136 (2010).
Pérez-Losada, M. A. R. C. O. S., Nolte, M. J., Crandall, K. A. & Shaw, P. W. Testing hypotheses of population structuring in the Northeast Atlantic Ocean and Mediterranean Sea using the common cuttlefish Sepia officinalis. Mol. Ecol. 16(13), 2667–2679 (2007).
Olaya-Restrepo, J., Erzini, K. & González-Wangüemert, M. Estimation of growth parameters for the exploited sea cucumber Holothuria arguinensis from South Portugal. Fish. Bull. 116(1), 1–8 (2018).
Taylor, N. G., Walters, C. J. & Martell, S. J. A new likelihood for simultaneously estimating von Bertalanffy growth parameters, gear selectivity, and natural and fishing mortality. Can. J. Fish. Aquat. Sci. 62(1), 215–223 (2005).
Pauly, D. On the interrelationships between natural mortality, growth parameters, and mean environmental temperature in 175 fish stocks. ICES J. Mar. Sci. 39(2), 175–192 (1980).
Source: Ecology - nature.com