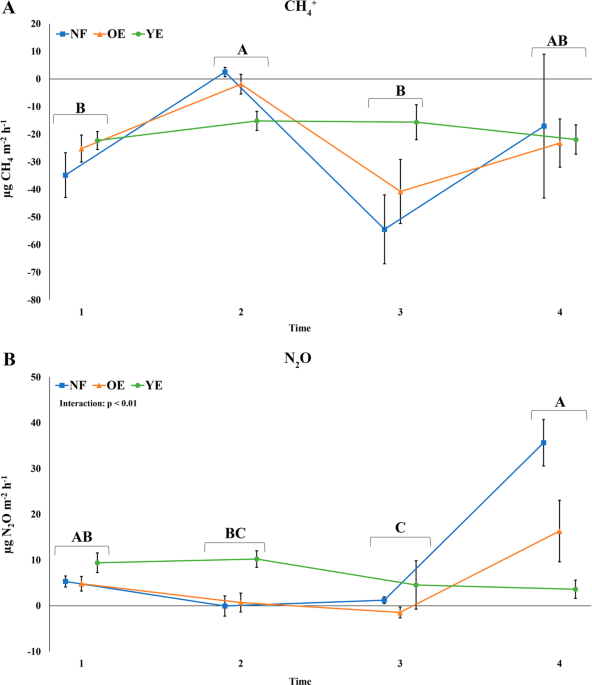
Cubasch, U. et al. Introduction. In Climate Change 2013: The Physical Science Basis. Contribution of Working Group I to the Fifth Assessment Report of the Intergovernmental Panel on Climate Change (ed. Intergovernmental Panel on Climate Change) 119–158, https://doi.org/10.1017/CBO9781107415324.007 (Cambridge University Press, 2013).
Hartmann, D. L. et al. Observations: Atmosphere and Surface. in Climate Change 2013: The Physical Science Basis. Contribution of Working Group I to the Fifth Assessment Report of the Intergovernmental Panel on Climate Change (ed. Intergovernmental Panel on Climate Change) 159–254, https://doi.org/10.1017/CBO9781107415324.008 (Cambridge University Press, 2013).
Gitay, H., Suárez, A. & Watson, R. Climate Change and Biodiversity. Intergovernmental Panel on Climate Change. Geneva (2002).
Myhre, G. et al. Anthropogenic and Natural Radiative Forcing. in Climate Change 2013: The Physical Science Basis. Contribution of Working Group I to the Fifth Assessment Report of the Intergovernmental Panel on Climate Change (ed. Change, I. P. on C.) 659–740 (Cambridge University Press, 2013).
Ravishankara, A. R., Daniel, J. S. & Portmann, R. W. Nitrous oxide (N2O): The dominant ozone-depleting substance emitted in the 21st century. Science (80-). 326, 123–125 (2009).
IPCC. Climate Change and Land: an IPCC special report on climate change, desertification, land degradation, sustainable land management, food security, and greenhouse gas fluxes in terrestrial ecosystems. In press (2019).
SEEG. Análise das Emissões Brasileiras de Gases de Efeito Estufa e suas implicações para as metas do Brasil – 1970–2018 (2019).
Climate Watch. Washington, DC: World Resources Institute, https://www.climatewatchdata.org/ (2018).
Food and Agriculture Organisation of the United Nations. Global Forest Resources Assessment 2015. How are the world’s forests changing?, http://www.fao.org/3/a-i4808e.pdf (2015).
Brazilian tree industry. Annual report of IBA (indústria brasileira de árvores) (2019).
Du, H. et al. Carbon Storage in a Eucalyptus Plantation Chronosequence in Southern China. Forests 6, 1763–1778 (2015).
Brancalion, P. H. S. et al. Exotic eucalypts: From demonized trees to allies of tropical forest restoration? J. Appl. Ecol. 00, 1–12 (2019).
Gonçalves, J. L. D. M. et al. Integrating genetic and silvicultural strategies to minimize abiotic and biotic constraints in Brazilian eucalypt plantations. For. Ecol. Manage. 301, 6–27 (2013).
Fest, B. J., Livesley, S. J., Drösler, M., van Gorsel, E. & Arndt, S. K. Soil-atmosphere greenhouse gas exchange in a cool, temperate Eucalyptus delegatensis forest in south-eastern Australia. Agric. For. Meteorol. 149, 393–406 (2009).
Livesley, S. J. et al. Soil-atmosphere exchange of greenhouse gases in a Eucalyptus marginata woodland, a clover-grass pasture, and Pinus radiata and Eucalyptus globulus plantations. Glob. Chang. Biol. 15, 425–440 (2009).
Martins, C. S. C., Nazaries, L., Macdonald, C. A., Anderson, I. C. & Singh, B. K. Water availability and abundance of microbial groups are key determinants of greenhouse gas fluxes in a dryland forest ecosystem. Soil Biol. Biochem. 86, 5–16 (2015).
Zhang, K. et al. Impact of nitrogen fertilization on soil-Atmosphere greenhouse gas exchanges in eucalypt plantations with different soil characteristics in southern China. Plos one 12, e0172142 (2017).
Cuer, C. A. et al. Short-term effect of Eucalyptus plantations on soil microbial communities and soil-atmosphere methane and nitrous oxide exchange. Sci. Rep. 8, 15133 (2018).
Dalal, R. C. & Allen, D. E. Greenhouse gas fluxes from natural ecosystems. Aust. J. Bot. 56, 369–407 (2008).
Laclau, J.-P. Nutrient Dynamics throughout the Rotation of Eucalyptus Clonal Stands in Congo. Ann. Bot. 91, 879–892 (2003).
Madsen, E. L. Microorganisms and their roles in fundamental biogeochemical cycles. Curr. Opin. Biotechnol. 22, 456–464 (2011).
Nazaries, L., Murrell, J. C., Millard, P., Baggs, L. & Singh, B. K. Methane, microbes and models: Fundamental understanding of the soil methane cycle for future predictions. Environ. Microbiol. 15, 2395–2417 (2013).
Signor, D. & Cerri, C. E. P. Nitrous oxide emissions in agricultural soils: a review. Pesqui. Agropecuária Trop. 43, 322–338 (2013).
Oertel, C., Matschullat, J., Zurba, K., Zimmermann, F. & Erasmi, S. Greenhouse gas emissions from soils — A review. Chemie der Erde – Geochemistry 76, 327–352 (2016).
Insam, H. & Wett, B. Control of GHG emission at the microbial community level. Waste Manag. 28, 699–706 (2008).
Alves, B. J. R. et al. Selection of the most suitable sampling time for static chambers for the estimation of daily mean N2O flux from soils. Soil Biol. Biochem. 46, 129–135 (2012).
Morais, R. F., Boddey, R. M., Urquiaga, S., Jantalia, C. P. & Alves, B. J. R. Ammonia volatilization and nitrous oxide emissions during soil preparation and N fertilization of elephant grass (Pennisetum purpureum Schum.). Soil Biol. Biochem. 64, 80–88 (2013).
Parada, A. E., Needham, D. M. & Fuhrman, J. A. Every base matters: assessing small subunit rRNA primers for marine microbiomes with mock communities, time series and global field samples. Environ. Microbiol. 18, 1403–1414 (2016).
Quince, C., Lanzen, A., Davenport, R. J. & Turnbaugh, P. J. Removing noise from pyrosequenced amplicons. BMC Bioinformatics 12(30), 1–18 (2011).
Schloss, P. D. et al. Introducing mothur: Open-source, platform-independent, community-supported software for describing and comparing microbial communities. Appl. Environ. Microbiol. 75, 7537–7541 (2009).
Quast, C. et al. The SILVA ribosomal RNA gene database project: improved data processing and web-based tools. Nucleic Acids Res. 41, D590–6 (2013).
Rognes, T., Flouri, T., Nichols, B., Quince, C. & Mahé, F. VSEARCH: a versatile open source tool for metagenomics. PeerJ 4, e2584 (2016).
Cole, J. R. et al. The Ribosomal Database Project: Improved alignments and new tools for rRNA analysis. Nucleic Acids Res. 37, 141–145 (2009).
Apprill, A., McNally, S., Parsons, R. & Weber, L. Minor revision to V4 region SSU rRNA 806R gene primer greatly increases detection of SAR11 bacterioplankton. Aquat. Microb. Ecol. 75, 129–137 (2015).
Amir, A. et al. Deblur Rapidly Resolves Single-Nucleotide Community Sequence Patterns. mSystems 2, 1–7 (2017).
Hammer, Ø., Harper, D. A. T. & Ryan, P. D. PAST: Paleontological statistics software package for education and data analysis. Palaeontol. Electron. 4, 1–9 (2001).
Dufrene, M. & Legendre, P. Species assemblages and indicator species: The need for a flexible asymmetrical approach. Ecol. Monogr. 67, 345–366 (1997).
McCune, B. & Mefford, M. J. PC-ORD v. 6.0. MjM Software, Gleneden Beach, OR (2010).
Liu, H. et al. Greenhouse gas fluxes from soils of different land-use types in a hilly area of South China. Agric. Ecosyst. Environ. 124, 125–135 (2008).
Yashiro, Y., Kadir, W. R., Okuda, T. & Koizumi, H. The effects of logging on soil greenhouse gas (CO2, CH4, N2O) flux in a tropical rain forest, Peninsular Malaysia. Agric. For. Meteorol. 148, 799–806 (2008).
Davidson, E. A. Fluxes of nitrous oxide and nitric oxide from terrestrial ecosystems. in Microbial Production and Consumption of Greenhouse Gases: Methane, Nitrogen Oxides and Halomethanes (eds. Rogers, J. & Whitman, W.) 219–235 (American Society of Microbiology, 1991).
Weslien, P., Klemedtsson, A. K., Börjesson, G. & Klemedtsson, L. Strong pH influence on N2O and CH4 fluxes from forested organic soils. Eur. J. Soil Sci. 60, 311–320 (2009).
Kunito, T. et al. Aluminum and acidity suppress microbial activity and biomass in acidic forest soils. Soil Biol. Biochem. 97, 23–30 (2016).
Illmer, P., Marschall, K. & Schinner, F. Influence of available aluminium on soil micro-organisms. Lett. Appl. Microbiol. 21, 393–397 (1995).
Flechard, C. R., Neftel, A., Jocher, M., Ammann, C. & Fuhrer, J. Bi-directional soil/atmosphere N2O exchange over two mown grassland systems with contrasting management practices. Glob. Chang. Biol. 11, 2114–2127 (2005).
Chapuis-Lardy, L., Wrage, N., Metay, A., Chotte, J.-L. & Bernoux, M. Soils, a sink for N2O? A review. Glob. Chang. Biol. 13, 1–17 (2007).
Chalk, P. M. & Smith, C. J. The role of agroecosystems in chemical pathways of N2O production. Agric. Ecosyst. Environ. 290, 106783 (2020).
Hazlett, P. W., Gordon, A. M., Voroney, R. P. & Sibley, P. K. Impact of harvesting and logging slash on nitrogen and carbon dynamics in soils from upland spruce forests in northeastern Ontario. 39, 43–57 (2007).
Liu, L. & Greaver, T. L. A review of nitrogen enrichment effects on three biogenic GHGs: The CO2 sink may be largely offset by stimulated N2O and CH4 emission. Ecol. Lett. 12, 1103–1117 (2009).
Aronson, E. L., Allison, S. D. & Helliker, B. R. Environmental impacts on the diversity of methane-cycling microbes and their resultant function. Front. Microbiol. 4, 1–15 (2013).
Navarrete, A. A. et al. Soil microbiome responses to the short-term effects of Amazonian deforestation. Mol. Ecol. 24, 2433–2448 (2015).
Crowther, T. W. et al. Predicting the responsiveness of soil biodiversity to deforestation: A cross-biome study. Glob. Chang. Biol. 20, 2983–2994 (2014).
Mendes, L. W. et al. Soil-Borne Microbiome: Linking Diversity to Function. Microb. Ecol. 70, 255–265 (2015).
Jangid, K. et al. Soil Biology & Biochemistry Land-use history has a stronger impact on soil microbial community composition than aboveground vegetation and soil properties. Soil Biol. Biochem. 43, 2184–2193 (2011).
Rachid, C. T. C. C. et al. Intercropped Silviculture Systems, a Key to Achieving Soil Fungal Community Management in Eucalyptus Plantations. Plos one 10, e0118515 (2015).
Pereira, A. P. D. A. et al. Shifts in the bacterial community composition along deep soil profiles in monospecific and mixed stands of Eucalyptus grandis and Acacia mangium. Plos one 12, e0180371 (2017).
Rachid, C. T. C. C. et al. Mixed plantations can promote microbial integration and soil nitrate increases with changes in the N cycling genes. Soil Biol. Biochem. 66, 146–153 (2013).
Lauber, C. L., Ramirez, K. S., Aanderud, Z., Lennon, J. & Fierer, N. Temporal variability in soil microbial communities across land-use types. ISME J. 7, 1641–1650 (2013).
Paula, F. S. et al. Land use change alters functional gene diversity, composition and abundance in Amazon forest soil microbial communities. Mol. Ecol. 23, 2988–2999 (2014).
Rachid, C. T. C. C. et al. Effect of sugarcane burning or green harvest methods on the Brazilian Cerrado soil bacterial community structure. Plos one 8, e59342 (2013).
O’Brien, S. L. et al. Spatial scale drives patterns in soil bacterial diversity. Environ. Microbiol. 18, 2039–2051 (2016).
Kroeger, M. E. et al. New Biological Insights Into How Deforestation in Amazonia Affects Soil Microbial Communities Using Metagenomics and Metagenome-Assembled Genomes. Front. Microbiol. 9, 1–13 (2018).
Ramirez, K. S. et al. Biogeographic patterns in below-ground diversity in New York City’s Central Park are similar to those observed globally. Proc. R. Soc. B 281, 20141988 (2014).
Lipson, D. A. Relationships between temperature responses and bacterial community structure along seasonal and altitudinal gradients. FEMS Microbiol. Ecol. 59, 418–427 (2007).
Campbell, C. A. et al. Seasonal trends in soil biochemical attributes: Effects of crop management on a Black Chernozem. Can. J. Soil Sci. 79, 85–97 (1999).
Cain, M. L., Subler, S., Evans, J. P. & Fortin, M.-J. Sampling spatial and temporal variation in soil nitrogen availability. Oecologia 118, 397–404 (1999).
Petersen, I. A. B., Meyer, K. M. & Bohannan, B. J. M. Meta-Analysis Reveals Consistent Bacterial Responses to Land Use Change Across the Tropics. Front. Ecol. Evol. 7, 1–9 (2019).
Rachid, C. T. et al. Physical-chemical and microbiological changes in Cerrado Soil under differing sugarcane harvest management systems. BMC Microbiol. 12, 170 (2012).
Wallis, P. D., Haynes, R. J., Hunter, C. H. & Morris, C. D. Effect of land use and management on soil bacterial biodiversity as measured by PCR-DGGE. Appl. Soil Ecol. 46, 147–150 (2010).
Marcondes de Souza, J. A., Carareto Alves, L. M., de Mello Varani, A. & de Macedo Lemos, E. G. The Family Bradyrhizobiaceae. In The Prokaryotes (eds. Rosenberg, E., DeLong, E. F., Lory, S., Stackebrandt, E. & Thompson, F.) 135–154, https://doi.org/10.1007/978-3-642-30197-1_253 (Springer Berlin Heidelberg, 2014).
Tamura, T., Ishida, Y., Nozawa, Y., Otoguro, M. & Suzuki, K.-I. Transfer of Actinomadura spadix Nonomura and Ohara 1971 to Actinoallomurus spadix gen. nov., comb. nov., and description of Actinoallomurus amamiensis sp. nov., Actinoallomurus caesius sp. nov., Actinoallomurus coprocola sp. nov., Actinoallomurus fulvus s. Int. J. Syst. Evol. Microbiol. 59, 1867–1874 (2009).
Bælum, J. et al. Direct analysis of tfdA gene expression by indigenous bacteria in phenoxy acid amended agricultural soil. ISME J. 2, 677–687 (2008).
Shannon, K. E. M. et al. Effect of nitrate and glucose addition on denitrification and nitric oxide reductase (cnorB) gene abundance and mRNA levels in Pseudomonas mandelii inoculated into anoxic soil. Antonie Van Leeuwenhoek 100, 183–195 (2011).
Yoshida, M., Ishii, S., Fujii, D., Otsuka, S. & Senoo, K. Identification of Active Denitrifiers in Rice Paddy Soil by DNA- and RNA-Based Analyses. Microbes Environ. 27, 456–461 (2012).
Morales, S. E., Cosart, T. & Holben, W. E. Bacterial gene abundances as indicators of greenhouse gas emission in soils. ISME J. 4, 799–808 (2010).
Lee, K. & Jose, S. Soil respiration, fine root production, and microbial biomass in cottonwood and loblolly pine plantations along a nitrogen fertilization gradient. For. Ecol. Manage. 185, 263–273 (2003).
Fisk, M. C. & Fahey, T. J. Microbial biomass and nitrogen cycling responses to fertilization and litter removal in young northern hardwood forests. Biogeochemistry 53, 201–223 (2001).
Meyer, K. M. et al. Conversion of Amazon rainforest to agriculture alters community traits of methane-cycling organisms. Mol. Ecol. 26, 1547–1556 (2017).
Prosser, J. I. & Nicol, G. W. Archaeal and bacterial ammonia-oxidisers in soil: the quest for niche specialisation and differentiation. Trends Microbiol. 20, 523–531 (2012).
Kerou, M. & Schleper, C. Nitrososphaera. in Bergey’s Manual of Systematics of Archaea and Bacteria 1–10 https://doi.org/10.1002/9781118960608.gbm01294 (John Wiley & Sons, Ltd. 2016).
Zhalnina, K. et al. Ca. Nitrososphaera and Bradyrhizobium are inversely correlated and related to agricultural practices in long-term field experiments. Front. Microbiol. 4, 1–13 (2013).
Yu, Y. et al. Effect of land use on the denitrification, abundance of denitrifiers, and total nitrogen gas production in the subtropical region of China. Biol. Fertil. Soils 50, 105–113 (2014).
Ducey, T. F. et al. Soil Physicochemical Conditions, Denitrification Rates, and Abundance in North Carolina Coastal Plain Restored Wetlands. J. Environ. Qual. 44, 1011 (2015).
Lammel, D. R., Nüsslein, K., Tsai, S. M. & Cerri, C. C. Land use, soil and litter chemistry drive bacterial community structures in samples of the rainforest and Cerrado (Brazilian Savannah) biomes in Southern Amazonia. Eur. J. Soil Biol. 66, 32–39 (2015).
Source: Ecology - nature.com