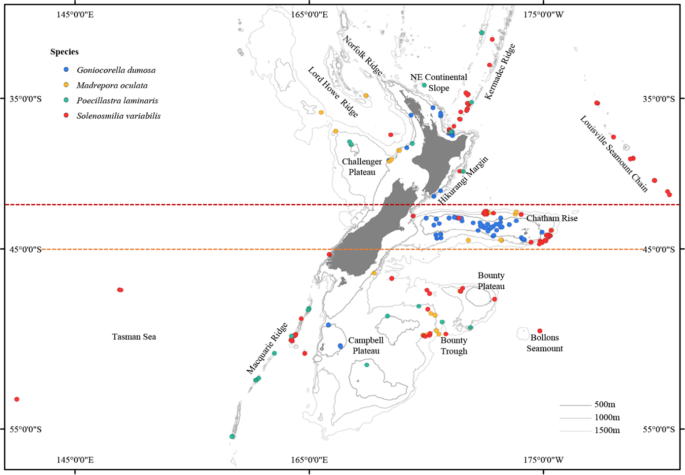
Hilário, A. et al. Estimating dispersal distance in the deep sea: Challenges and applications to marine reserves. Front. Mar. Sci. 2, 6 (2015).
Baco, A. R. et al. A synthesis of genetic connectivity in deep-sea fauna and implications for marine reserve design. Mol. Ecol. 25, 3276–3298 (2016).
Zeng, C., Rowden, A. A., Clark, M. R. & Gardner, J. P. A. Population genetic structure and connectivity of deep-sea stony corals (Order Scleractinia) in the New Zealand region: Implications for the conservation and management of vulnerable marine ecosystems. Evol. Appl. 10, 1040–1054 (2017).
Amils, R., Ellis-Evans, C., Hinghofer-Szalkay, H., Rothschild, L. J. & Mancinelli, R. L. Life in extreme environments. Nature 409, 1092–1101 (2001).
Miller, K. J., Williams, A., Rowden, A. A., Knowles, C. & Dunshea, G. Conflicting estimates of connectivity among deep-sea coral populations. Mar. Ecol. 31, 144–157 (2010).
Bors, E. K., Rowden, A. A., Maas, E. W., Clark, M. R. & Shank, T. M. Patterns of deep-sea genetic connectivity in the New Zealand region: Implications for management of benthic ecosystems. PLoS One 7, e49474 (2012).
Miller, K.J. & Gunasekera, R. A comparison of genetic connectivity in two deep sea corals to examine whether seamounts are isolated islands or stepping stones for dispersal. Sci. Rep. 7, (2017).
Chevaldonné, P., Joilivet, D., Vangriesheim, A. & Desbruyéres, D. Hydrothermal-vent alvinellid polychaete dispersal in the eastern Pacific. 1. Influence of vent site distribution, bottom currents, and biological patterns. Limnol. Oceanogr. 42, 67–80 (1997).
Arellano, S. M., Van Gaest, A. L., Johnson, S. B., Vrijenhoek, R. C. & Young, C. M. Larvae from deep-sea methane seeps disperse in surface waters. Proc. R. Soc. B 281, 20133276 (2014).
Miller, K., Rowden, A., Williams, A. & Häussermann, V. Out of their depth? Isolated deep populations of the cosmopolitan coral Desmophyllum dianthus may be highly vulnerable to environmental change. PLoS One 6, e19004 (2011).
Baco, A. R. & Cairns, S. D. Comparing molecular variation to morphological species designations in the deep-sea coral narella reveals new insights into seamount coral ranges. PLoS One 7, e45555 (2012).
Catarino, D. et al. The Pillars of Hercules as a bathymetric barrier to gene flow promoting isolation in a global deep-sea shark (Centroscymnus coelolepis). Mol. Ecol. 24, 6061–6079 (2015).
Ramirez-Llodra, E. et al. Man and the last great wilderness: Human impact on the deep sea. PLoS One 6, e22588 (2011).
Zeng, C., Clark, M.R., Rowden, A.A. & Gardner, J. P. A. The use of spatially explicit genetic variation data from four deep-sea sponges to inform the protection of Vulnerable Marine Ecosystems. Sci. Rep. (2019).
Taylor, M. L. & Roterman, C. N. Invertebrate population genetics across Earth’s largest habitat: The deep-sea floor. Mol. Ecol. 26, 4872–4896 (2017).
FAO. International guidelines for the management of deep-sea fisheries in the high seas. https://doi.org/10.1007/s13398-014-0173-7.2 (2009).
Aguilar, R., Perry, A. L. & López, J. Conservation and management of vulnerable marine benthic ecosystems. in Marine animal forests: The ecology of benthic biodiversity hotspots (eds. Rossi, S., Bramanti, L., Gori, A. & Covadonga, O.) 1–43 (Springer International Publishing, 2017). https://doi.org/10.1007/978-3-319-17001-5_34-1.
Adkins, J. F., Boyle, E. A., Curry, W. B. & Lutringer, A. Stable isotopes in deep-sea corals and a new mechanism for ‘vital effects’. Geochim. Cosmochim. Acta 67, 1129–1143 (2003).
Kiriakoulakis, K. et al. Lipids and nitrogen isotopes of two deep-water corals from the North-East Atlantic: Initial results and implications for their nutrition. in Cold-water Corals and Ecosystems 715–729, https://doi.org/10.1007/3-540-27673-4_37 (2005).
Davies, A. J., Wisshak, M., Orr, J. C. & Murray Roberts, J. Predicting suitable habitat for the cold-water coral Lophelia pertusa (Scleractinia). Deep. Res. Part I Oceanogr. Res. Pap. 55, 1048–1062 (2008).
Dullo, W. C., Flögel, S. & Rüggeberg, A. Cold-water coral growth in relation to the hydrography of the Celtic and Nordic European continental margin. Mar. Ecol. Prog. Ser. 371, 165–176 (2008).
Dodds, L. A., Black, K. D., Orr, H. & Roberts, J. M. Lipid biomarkers reveal geographical differences in food supply to the cold-water coral Lophelia pertusa (Scleractinia). Mar. Ecol. Prog. Ser. 397, 113–124 (2009).
Guinan, J., Grehan, A. J., Dolan, M. F. J. & Brown, C. Quantifying relationships between video observations of cold-water coral cover and seafloor features in rockall trough, west of Ireland. Mar. Ecol. Prog. Ser. 375, 125–138 (2009).
Roberts, J. M. et al. Mingulay reef complex: An interdisciplinary study of cold-water coral habitat, hydrography and biodiversity. Mar. Ecol. Prog. Ser. 397, 139–151 (2009).
Case, D. H., Robinson, L. F., Auro, M. E. & Gagnon, A. C. Environmental and biological controls on Mg and Li in deep-sea scleractinian corals. Earth Planet. Sci. Lett. 300, 215–225 (2010).
Dolan, M. F. J., Grehan, A. J., Guinan, J. C. & Brown, C. Modelling the local distribution of cold-water corals in relation to bathymetric variables: Adding spatial context to deep-sea video data. Deep. Res. Part I Oceanogr. Res. Pap. 55, 1564–1579 (2008).
Brooke, S. D., Holmes, M. W. & Young, C. M. Sediment tolerance of two different morphotypes of the deep-sea coral Lophelia pertusa from the Gulf of Mexico. Mar. Ecol. Prog. Ser. 390, 137–144 (2009).
Frederiksen, R., Jensen, A. & Westerberg, H. The distribution of the scleractinian coral Lophelia pertusa around the Faroe Islands and the relation to internal tidal mixing. Sarsia 77, 157–171 (1992).
McCulloch, M. et al. Resilience of cold-water scleractinian corals to ocean acidification: Boron isotopic systematics of pH and saturation state up-regulation. Geochim. Cosmochim. Acta 87, 21–34 (2012).
Manel, S., Schwartz, M. K., Luikart, G. & Taberlet, P. Landscape genetics: Combining landscape ecology and population genetics. Trends Ecol. Evol. 18, 189–197 (2003).
Richardson, J. L., Brady, S. P., Wang, I. J. & Spear, S. F. Navigating the pitfalls and promise of landscape genetics. Mol. Ecol. 25, 849–863 (2016).
Selkoe, K. A., Henzler, C. M. & Gaines, S. D. Seascape genetics and the spatial ecology of marine populations. Fish Fish. 9, 363–377 (2008).
Riginos, C. & Liggins, L. Seascape genetics: Populations, individuals, and genes marooned and adrift. Geogr. Compass 7, 197–216 (2013).
Selkoe, K. A. et al. A decade of seascape genetics: Contributions to basic and applied marine connectivity. Mar. Ecol. Prog. Ser. 554, 1–19 (2016).
Crandall, E. D., Treml, E. A. & Barber, P. H. Coalescent and biophysical models of stepping-stone gene flow in neritid snails. Mol. Ecol. 21, 5579–5598 (2012).
Liggins, L., Treml, E. A., Possingham, H. P. & Riginos, C. Seascape features, rather than dispersal traits, predict spatial genetic patterns in co-distributed reef fishes. J. Biogeogr. 43, 256–267 (2016).
Silva, C. N. S. & Gardner, J. P. A. Identifying environmental factors associated with the genetic structure of the New Zealand scallop: Linking seascape genetics and ecophysiological tolerance. ICES J. Mar. Sci. 73, 1925–1934 (2016).
Wei, K., Wood, A. R. & Gardner, J. P. A. Seascape genetics of the New Zealand greenshell mussel: Sea surface temperature explains macrogeographic scale genetic variation. Mar. Ecol. Prog. Ser. 477, 107–121 (2013).
Rousset, F. Genetic differentiation and estimation of gene flow from F-statistics under isolation by distance. Genetics 154, 1219–1228 (1997).
Holderegger, R., Kamm, U. & Gugerli, F. Adaptive vs. neutral genetic diversity: Implications for landscape genetics. Landsc. Ecol. 21, 797–807 (2006).
Wei, K., Wood, A. R. & Gardner, J. P. A. Population genetic variation in the New Zealand greenshell mussel: Locus-dependent conflicting signals of weak structure and high gene flow balanced against pronounced structure and high self-recruitment. Mar. Biol. 160, 931–949 (2013).
Watling, L., Guinotte, J., Clark, M. R. & Smith, C. R. A proposed biogeography of the deep ocean floor. Prog. Oceanogr. 111, 91–112 (2013).
Chiswell, S. M., Bostock, H. C., Sutton, P. J. & Williams, M. J. Physical oceanography of the deep seas around New Zealand: A review. New Zeal. J. Mar. Freshw. Res. 49, 1–32 (2015).
Bucklin, A., Wilson, R. R. & Smith, K. L. Genetic differentiation of seamount and basin populations of the deep-sea amphipod Eurythenes gryllus. Deep Sea Res. Part A, Oceanogr. Res. Pap. 34, 1795–1810 (1987).
Yang, C. H. et al. Connectivity of the squat lobsters Shinkaia crosnieri (Crustacea: Decapoda: Galatheidae) between cold seep and hydrothermal vent habitats. Bull. Mar. Sci. 32, 257 (2015).
Waller, R. G. & Tyler, P. A. The reproductive biology of two deep-water, reef-building scleractinians from the NE Atlantic Ocean. Coral Reefs 24, 514–522 (2005).
Waller, R. G. Deep-water Scleractinia (Cnidaria: Anthozoa): Current knowledge of reproductive processes. Cold-water Corals Ecosyst. 691–700, https://doi.org/10.1007/3-540-27673-4_35 (2005).
Waller, R. G., Tyler, P. A. & Gage, J. D. Sexual reproduction in three hermaphroditic deep-sea Caryophyllia species (Anthozoa: Scleractinia) from the NE Atlantic Ocean. Coral Reefs 24, 594–602 (2005).
Pires, D. O., Silva, J. C. & Bastos, N. D. Reproduction of deep-sea reef-building corals from the southwestern. Atlantic. Deep. Res. Part II Top. Stud. Oceanogr. 99, 51–63 (2014).
Burgess, S. N. & Babcock, R. C. In Cold-water Corals and Ecosystems. (eds Freiwald, A. & Roberts, J. M.) 701–713 (Springer-verlag 2015).
Galindo, H. M. et al. Seascape genetics along a steep cline: Using genetic patterns to test predictions of marine larval dispersal. Mol. Ecol. https://doi.org/10.1111/j.1365-294X.2010.04694.x (2010).
Nanninga, G. B., Saenz-Agudelo, P., Manica, A. & Berumen, M. L. Environmental gradients predict the genetic population structure of a coral reef fish in the Red Sea. Mol. Ecol. https://doi.org/10.1111/mec.12623 (2014).
Saha, A. et al. Seascape genetics of saithe (Pollachius virens) across the North Atlantic using single nucleotide polymorphisms. ICES J. Mar. Sci. 72, 2732–2741 (2015).
Silva, C. N. S. & Gardner, J. P. A. Emerging patterns of genetic variation in the New Zealand endemic scallop Pecten novaezelandiae. Mol. Ecol. 24, 5379–5393 (2015).
Durrant, H. M. S., Barrett, N. S., Edgar, G. J., Coleman, M. A. & Burridge, C. P. Seascape habitat patchiness and hydrodynamics explain genetic structuring of kelp populations. Mar. Ecol. Prog. Ser. 587, 81–92 (2018).
Boschen, R. E., Rowden, A. A., Clark, M. R. & Gardner, J. P. A. Limitations in the use of archived vent mussel samples to assess genetic connectivity among seafloor massive sulfide deposits: A case study with implications for environmental management. Front. Mar. Sci. 2, 1–14 (2015).
Bryan, T. L. & Metaxas, A. Distribution of deep-water corals along the North American continental margins: Relationships with environmental factors. Deep. Res. Part I Oceanogr. Res. Pap. 53, 1865–1879 (2006).
Tittensor, D. P. et al. Predicting global habitat suitability for stony corals on seamounts. J. Biogeogr. 36, 1111–1128 (2009).
Woodby, D., Carlile, D. & Hulbert, L. Predictive modeling of coral distribution in the central Aleutian Islands, USA. Mar. Ecol. Prog. Ser. 397, 227–240 (2009).
Fisher, C. R. et al. Footprint of deepwater horizon blowout impact to deep-water coral communities. Proc. Natl. Acad. Sci. USA 111, 11744–9 (2014).
Tracey, D. M., Rowden, A. A., Mackay, K. A. & Compton, T. Habitat-forming cold-water corals show affinity for seamounts in the New Zealand region. Mar. Ecol. Prog. Ser. 430, 1–22 (2011).
Thiem, Ø., Ravagnan, E., Fosså, J. H. & Berntsen, J. Food supply mechanisms for cold-water corals along a continental shelf edge. J. Mar. Syst. https://doi.org/10.1016/j.jmarsys.2005.12.004 (2006).
White, M., Bashmachnikov, I., Arístegui, J. & Martins, A. Physical processes and seamount productivity. in Seamounts: Ecology, fisheries & conservation (eds. Pitcher, T. J. et al.) 10.1002/9780470691953.ch4. (Blackwell Publishing Ltd, 2008).
Guinotte, J. M. et al. Will human-induced changes in seawater chemistry alter the distribution of deep-sea scleractinian corals? Front. Ecol. Environ. 4, 141–146 (2006).
Davies, A. J. & Guinotte, J. M. Global habitat suitability for framework-forming cold-water corals. PLoS One 6, e18483 (2011).
Hurst, R., Renwick, J., Uddstrom, M., Kennan, S. & Rickard, G. J. Climate and ocean trends of potential relevance to fisheries in the New Zealand region.New Zealand Aquatic Environment and Biodiversity Report No. 90. (2012).
Abelson, A., Miloh, T. & Loya, Y. Flow patterns induced by substrata and body morphologies of benthic organisms, and their roles in determining availability of food particles. Limnol. Oceanogr. 38, 1116–1124 (1993).
Kaiser, M. J. et al. Marine ecology: Processes, systems and impacts. Marine Ecology vol. 0 (Oxford University Press, 2011).
Thorson, G. Reproductive and larval ecology of marine bottom invertebrates. Biol. Rev. 25, 1–45 (1950).
Buss, L. W. & Jackson, J. B. C. Planktonic food availability and suspension-feeder abundance: Evidence of in situ depletion. J. Exp. Mar. Bio. Ecol. 49, 151–161 (1981).
Bertness, M. D. & Gaines, S. D. Larval dispersal and local adaptation in acorn barnacles. Evolution (N. Y). 47, 316–320 (1993).
Qian, P. Y. Effect of food quantity on growth and reproductive characteristics of Capitella sp (Annelida: Polychaeta). Invertebr. Reprod. Dev. 26, 175–185 (1994).
Pechenik, J. A., Estrella, M. S. & Hammer, K. Food limitation stimulates metamorphosis of competent larvae and alters postmetamorphic growth rate in the marine prosobranch gastropod Crepidula fornicata. Mar. Biol. 127, 267–275 (1996).
Fouzai, N., Opdal, A., Jørgensen, C. & Fiksen, Ø. Effects of temperature and food availability on larval cod survival: A model for behaviour in vertical gradients. Mar. Ecol. Prog. Ser. 529, 199–212 (2015).
Seibel, B. A. & Drazen, J. C. The rate of metabolism in marine animals: Environmental constraints, ecological demands and energetic opportunities. Philos. Trans. R. Soc. Lond. B. Biol. Sci. 362, 2061–78 (2007).
Giles, E. C., Saenz-Agudelo, P., Hussey, N. E., Ravasi, T. & Berumen, M. L. Exploring seascape genetics and kinship in the reef sponge Stylissa carteri in the Red Sea. Ecol. Evol. 5, 2487–2502 (2015).
Hadas, E., Shpigel, M. & Ilan, M. Particulate organic matter as a food source for a coral reef sponge. J. Exp. Biol. 212, 3643–3650 (2009).
Knudby, A., Kenchington, E. & Murillo, F. J. Modeling the distribution of Geodia sponges and sponge grounds in the Northwest Atlantic. PLoS One 8, e82306 (2013).
Rooper, C. N., Zimmermann, M., Prescott, M. M. & Hermann, A. J. Predictive models of coral and sponge distribution, abundance and diversity in bottom trawl surveys of the Aleutian Islands, Alaska. Mar. Ecol. Prog. Ser. 503, 157–176 (2014).
Waples, R. S. & Do, C. ldne: A program for estimating effective population size from data on linkage disequilibrium. Mol. Ecol. Resour. 8, 753–6 (2008).
McCook, L. J. et al. Management under uncertainty: Guide-lines for incorporating connectivity into the protection of coral reefs. Coral Reefs 28, 353–366 (2009).
Haig, S. M. et al. The conservation genetics juggling act: Integrating genetics and ecology, science and policy. Evol. Appl. 9, 181–195 (2016).
Selkoe, K. A. et al. The DNA of coral reef biodiversity: Predicting and protecting genetic diversity of reef assemblages. Proc. R. Soc. B Biol. Sci. 283, 20160354 (2016).
Anderson, O. F. et al. Field validation of habitat suitability models for vulnerable marine ecosystems in the South Pacific Ocean: Implications for the use of broad-scale models in fisheries management. Ocean Coast. Manag. 120, 110–126 (2016).
Anderson, O. F. et al. Habitat suitability models for predicting the occurrence of vulnerable marine ecosystems in the seas around New Zealand. Deep. Res. Part I Oceanogr. Res. Pap. 115, 265–292 (2016).
Boschen, R. E. et al. A primer for use of genetic tools in selecting and testing the suitability of set-aside sites protected from deep-sea seafloor massive sulfide mining activities. Ocean Coast. Manag. 122, 37–48 (2016).
Prunier, J. G. et al. Optimizing the trade-off between spatial and genetic sampling efforts in patchy populations: Towards a better assessment of functional connectivity using an individual-based sampling scheme. Mol. Ecol. 22, 5516–5530 (2013).
Hall, L. A. & Beissinger, S. R. A practical toolbox for design and analysis of landscape genetics studies. Landsc. Ecol. 29, 1487–1504 (2014).
Luximon, N., Petit, E. J. & Broquet, T. Performance of individual vs. group sampling for inferring dispersal under isolation-by-distance. Mol. Ecol. Resour. 14, 745–752 (2014).
Gebremedhin, B. et al. Frontiers in identifying conservation units: From neutral markers to adaptive genetic variation. Anim. Conserv. 12, 107–109 (2009).
Antao, T., Lopes, A., Lopes, R. J., Beja-Pereira, A. & Luikart, G. LOSITAN: A workbench to detect molecular adaptation based on a Fst-outlier method. BMC Bioinformatics 9, 1–5 (2008).
van Oosterhout, C., Hutchinson, W. F., Wills, D. P. M. & Shipley, P. MICRO-CHECKER: Software for identifying and correcting genotyping errors in microsatellite data. Mol. Ecol. Notes 4, 535–538 (2004).
Gagnaire, P. A. et al. Using neutral, selected, and hitchhiker loci to assess connectivity of marine populations in the genomic era. Evol. Appl. 8, 769–786 (2015).
Graham, M. H. Confronting multicollinearity in ecological multiple regression. Ecology 84, 2809–2815 (2003).
Team, R. C. R. A Language and Environment for Statistical Computing. Vienna, Austria (2019).
Peakall, R. & Smouse, P. E. GenALEx 6.5: Genetic analysis in Excel. Population genetic software for teaching and research-an update. Bioinformatics 28, 2537–2539 (2012).
Anderson, M. J., Gorley, R. N. & Clarke, K. R. PERMANOVA+ for PRIMER: Guide to Software and Statistical Methods. (primer-E Limited, 2008).
Source: Ecology - nature.com