The results of the current research at 1000 K are in significant agreement with the results of previous investigations16,17. All hydrocarbons produced from methane in DAC by Kolesnikov, et al.17 were detected in our product mixture synthesized at 1000(±25) K and 2.5(±0.2) GPa: ethane, propane, n-butane and graphite. Iso-butane may also be present in the products mixture in the DAC experiments; however, its detection may be difficult due to the similar Raman signals for propane, butane and i-butane24,25. The trace amount of pentane and hexane isomers in our products mixture was detected by virtue of the large volume of the sample and the high sensitivity of the gas chromatography equipment.
The results of the current research support the hypothesis about the “methane path” mechanism of hydrocarbons synthesis from inorganic donors of carbon and hydrogen at extreme thermobaric conditions through the stage of methane formation11,26. The abiogenic synthesis of hydrocarbons was carried out in the large high-pressure unit “KONAK” with analysis by gas chromatography. Methane and heavier hydrocarbons were formed from CaCO3 and H2O in the presence of iron compounds at a wide range of thermobaric conditions (up to 11 GPa and 1800 K). The composition of normal and iso-alkanes up to C6H14, detected in the product mixture by gas chromatography combined with mass spectrometry, is similar to the hydrocarbon systems, produced from methane in our experiments.
A significant increase in the duration of the heating in our experiments compared to the 10 s exposure of the previous experiments17,27 did not drastically change the composition of the reaction products produced at similar pressure and temperature. However, the further increasing in exposure time leaded to the growth of heavy hydrocarbons (pentane and hexane isomers) in the product mixture (Fig. 1). The relative amount of ethane, propane, and butanes was kept almost constant in the series of experiments at 1000 K and 2.5 GPa with 4 hours and 10 hours of exposure time, while the amount of pentane and hexane isomers slightly grew. It contradicts the hypothesis that chemical equilibrium is reached very rapidly, however, the formation of heavier hydrocarbons from methane occurs instantaneously27.
The total amount of ethane, propane and butanes is more than 25% volume in the gaseous products synthesized at 1000(±25) K and 2.5(±0.2) GPa, thus making the composition of the “equilibrium” hydrocarbon system similar to “wet” natural gas (Table 2, Fig. 4b).
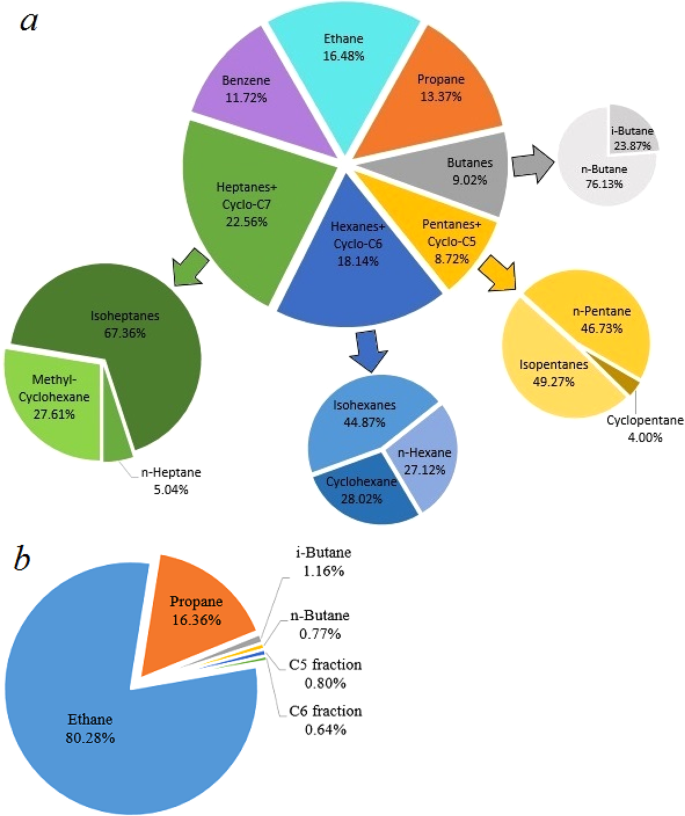
Composition of the heating product mixture (methane excluded): (a) formed at 850(±25) K and 2.5(±0.2) GPa during 10 hours, (b) formed at 1000(±25) K and 2.5(±0.2) GPa during 10 hours.
At a lower temperature (850(±25) K), a complex hydrocarbon mixture (up to seven carbon atoms in composition) was produced from methane. Similar to the series of experiments at 1000(±25) K and 2.5(±0.2) GPa, methane predominated in the product mixture. In addition to the normal alkanes, new classes of hydrocarbons were formed from methane: iso-alkanes, naphthenes and aromatics. All the isomers of alkanes from butane to heptane were detected by gas chromatography.
Figure 4 shows the composition of the gaseous products (methane is excluded) generated from methane after 10 hours of heating at 850(±25) K and 2.5(±0.2) GPa and at 1000(±25) K and 2.5(±0.2) GPa. The product mixture consists of light components of petroleum (Fig. 4a). The scheme of possible pathways of heavier hydrocarbons formation is presented in Fig. 5. The synthesis of heavier hydrocarbons is carried out via the radical mechanism28 focused mostly on the growth of the carbon-carbon bonds, isomerization and cyclization. Unsaturated hydrocarbons, which were also detected by Raman spectroscopy in the DAC experiments at similar thermobaric conditions29, may be the intermediate components due to their trace amount in the product mixture. One of the possible explanation is the deficiency of hydrogen in the reaction system that may lead to the formation of unsaturated hydrocarbons. In the complex hydrocarbon mixture produced from methane at 850(±25) K and 2.5(±0.2) GPa (Table 1), n-alkanes predominate for butane and pentane fractions in the experiments with time exposure of 0.5 and 2 hours. However, iso-alkanes prevailed in the experiments with more extensive heating (4 and 10 hours) due to the intensification of isomerization reactions28. Higher thermal stability of iso-structure can be explained by the more energetically stable and three-dimensionally substantial branched structure of large hydrocarbon molecules. The same situation takes place in the product mixtures produced from methane at 1000(±25) K and 2.5(±0.2) GPa: the relative amount of i-butane increases in the system after 10 hours heating.
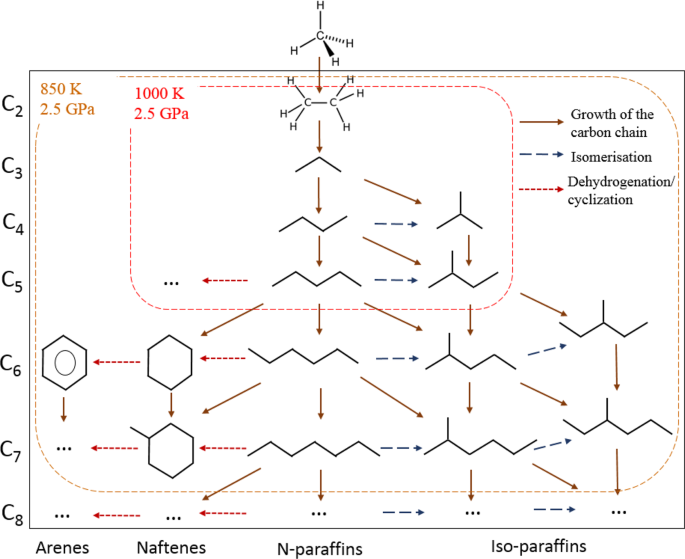
The scheme of methane transformations pathways. Solid brown arrow – reactions with the growth of the carbon-carbon chain, blue dashed line – isomerization of the synthesized hydrocarbon, red dashed line – dehydrogenation with the formation of the cycle chain or aromatic chain.
Our experiments describe the possible chemical transformations of methane in the C-O-H fluid at thermobaric conditions corresponding to the upper depth border of the abiogenic hydrocarbons formation zone of 70–80 km20,30. Methane, generated from the inorganic compounds in this mantle area or transported to this zone from the deeper level of the asthenosphere by the deep fluid31 can be transformed into heavier hydrocarbons. The complex hydrocarbon mixtures, generated in the upper mantle from methane, can migrate to the Earth’s crust through deep faults31 or in subduction zones along the weakened surface of the slab32 and contribute to petroleum deposits.
Our results indicate that at 2.5 GPa the temperature limit for heavier hydrocarbons C6+ is somewhere between 850 K and 1000 K. We cannot suggest what are the depth limits of the thermobaric stability zone for complex hydrocarbons mixtures, however, we suppose that at higher pressure the temperature limit for heavier hydrocarbons C6+ may be higher. As a result, it is expected that the existence of complex hydrocarbon mixtures is not limited by the depth of 70–80 km, but it is governed by the still unknown pressure-temperature correlation in the mantle.
It was strongly considered that methane was the predominant hydrocarbon component in the mantle fluids, and because of this hypothesis only methane33,34 and sometimes methane with ethane27) were taken into consideration in the C-O-H the mantle fluid modelling. However, our experiments suggest that a significant part of methane could be transformed into heavier hydrocarbons at the thermobaric conditions of the upper mantle (Tables 1, 2). Therefore, at least in the mantle zones with thermobaric conditions, compatible to ones, modelled in our experiments, it is expected that complex hydrocarbon mixtures may exist and, therefore, should be included in the C-O-H fluid modelling. The possible existence of heavy hydrocarbons in the mantle is supported by the literature data about the hydrocarbon inclusions in the mantle derived xenoliths. The deep mantle xenoliths, observed in various alkaline basic and ultrabasic igneous rocks, are one of the most important sources of information about the nature of the upper mantle. Matson, et al.35 studied inclusions in amphiboles from the mantle xenoliths selected in Vulcan’s Throne (United States). These amphiboles contain CH4, C2H4, C3H8, and the heavier hydrocarbons. Methane concentrations vary from 200 to 500 g/t. According to experiments, amphibole-bearing xenoliths crystallize at the depth of 65 km.
Source: Ecology - nature.com